Select The Five Major Mechanisms Of Antimicrobial Resistance
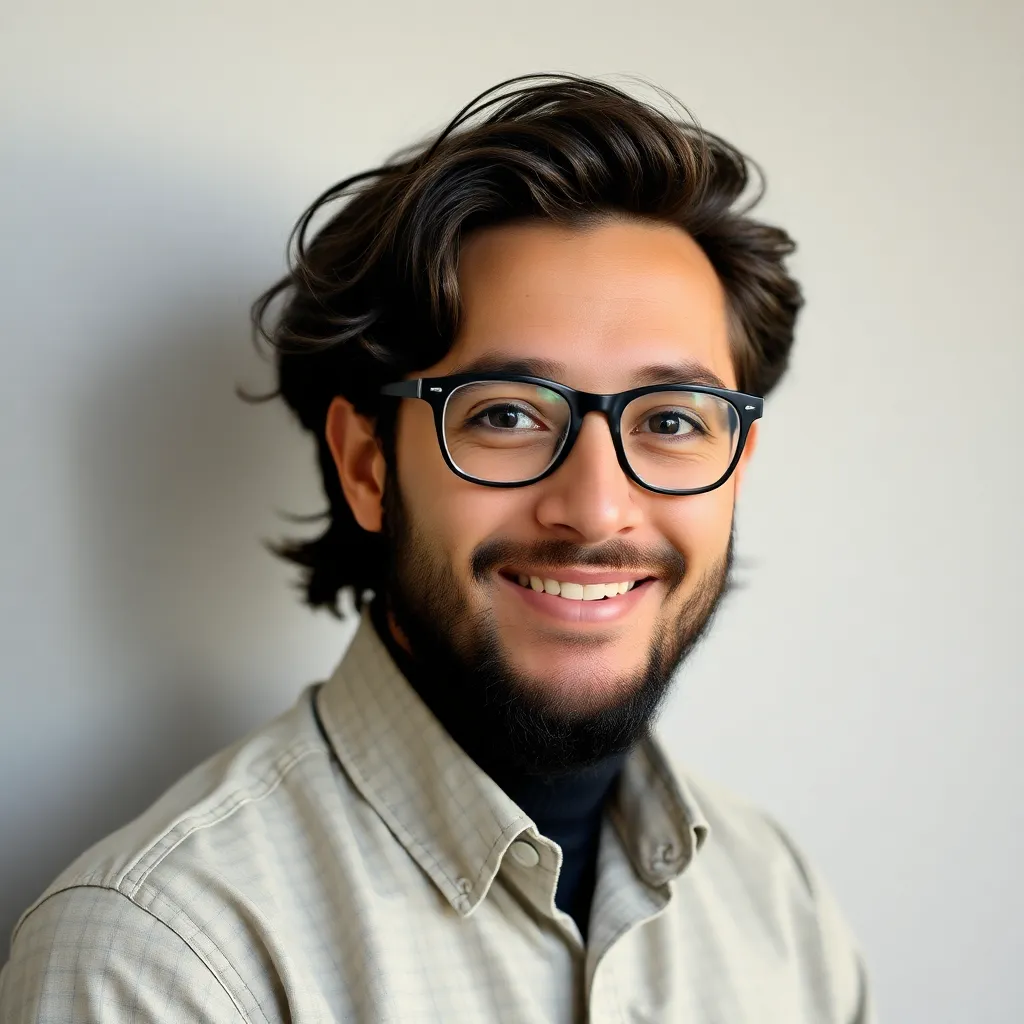
Holbox
Mar 30, 2025 · 7 min read

Table of Contents
- Select The Five Major Mechanisms Of Antimicrobial Resistance
- Table of Contents
- Five Major Mechanisms of Antimicrobial Resistance: A Deep Dive
- 1. Target Modification: Changing the Lock
- Examples of Target Modification:
- 2. Enzymatic Inactivation: Destroying the Key
- Examples of Enzymatic Inactivation:
- 3. Reduced Drug Accumulation: Preventing Entry
- Examples of Reduced Drug Accumulation:
- 4. Target Overproduction/Bypass: Overcoming Inhibition
- Examples of Target Overproduction/Bypass:
- 5. Efflux Pumps: The Cellular Vacuum Cleaners
- Characteristics of Efflux Pumps:
- Conclusion: A Multifaceted Challenge
- Latest Posts
- Latest Posts
- Related Post
Five Major Mechanisms of Antimicrobial Resistance: A Deep Dive
Antimicrobial resistance (AMR) is a global health crisis, threatening our ability to treat common infections with antibiotics, antifungals, antivirals, and antiparasitics. Understanding the mechanisms driving this resistance is crucial for developing effective strategies to combat it. This article delves into the five major mechanisms of antimicrobial resistance: target modification, enzymatic inactivation, reduced drug accumulation, target overproduction/bypass, and efflux pumps.
1. Target Modification: Changing the Lock
This mechanism involves altering the structure of the antimicrobial drug's target site within the microbe. This effectively changes the "lock" so that the "key" (the drug) no longer fits. This alteration prevents the drug from binding and exerting its effect.
Examples of Target Modification:
- Ribosomal mutations: Many antibiotics target bacterial ribosomes, the protein synthesis machinery. Mutations in ribosomal genes can alter the ribosome's structure, preventing antibiotic binding. This is commonly seen with resistance to aminoglycosides, macrolides, and tetracyclines. Specific mutations can impact the drug binding site or its efficacy, leading to varying degrees of resistance.
- Enzyme mutations: Some antibiotics target specific bacterial enzymes crucial for metabolism or cell wall synthesis. Mutations in the genes encoding these enzymes can lead to structural changes, rendering the enzyme less susceptible to the antibiotic. This is observed in resistance to penicillin and its derivatives. Mutations can reduce the drug’s affinity for its target or altogether prevent binding.
- DNA gyrase mutations: Quinolones target bacterial DNA gyrase and topoisomerase IV, enzymes essential for DNA replication. Mutations in the genes encoding these enzymes can reduce the drug's binding affinity, leading to quinolone resistance. These mutations are often associated with specific amino acid changes in the enzyme's active site. The location and nature of the mutations significantly impact the level of resistance.
- Penicillin-binding protein (PBP) mutations: Penicillins and cephalosporins target bacterial PBPs involved in peptidoglycan synthesis. Mutations in the pbp genes can alter the PBP's structure, reducing the antibiotic's binding affinity. This commonly leads to resistance against beta-lactam antibiotics. These mutations can result in altered antibiotic binding and reduced enzyme inhibition, ultimately leading to reduced susceptibility to the drug.
Significance: Target modification is a prevalent mechanism of resistance, often leading to cross-resistance – resistance to multiple drugs that target the same site. This poses a significant challenge in treatment.
2. Enzymatic Inactivation: Destroying the Key
Enzymatic inactivation involves the production of enzymes by the microbe that chemically modify or degrade the antibiotic, rendering it ineffective. This is akin to breaking the "key" before it can reach the "lock."
Examples of Enzymatic Inactivation:
- Beta-Lactamase production: Many bacteria produce beta-lactamases, enzymes that hydrolyze the beta-lactam ring of penicillin and cephalosporin antibiotics. This destroys the antibiotic's activity. Extended-spectrum beta-lactamases (ESBLs) can inactivate a wide range of beta-lactam antibiotics, posing a serious clinical challenge. The production of these enzymes is often encoded by plasmids, facilitating their spread among bacterial populations.
- Aminoglycoside-modifying enzymes (AMEs): These enzymes modify aminoglycoside antibiotics by adding chemical groups (acetylation, adenylation, or phosphorylation), preventing them from binding to their ribosomal target. This mechanism is responsible for widespread resistance to aminoglycosides. These enzymes are frequently plasmid-encoded and are known to confer resistance against multiple aminoglycosides.
- Chloramphenicol acetyltransferase (CAT): This enzyme acetylates chloramphenicol, reducing its binding to the bacterial ribosome and rendering it inactive. The gene encoding CAT is often located on plasmids, allowing for horizontal transfer of resistance. This enzymatic inactivation effectively prevents the drug from reaching its target site.
Significance: Enzymatic inactivation is a highly efficient mechanism, often conferring high levels of resistance. The widespread dissemination of genes encoding these enzymes, especially via plasmids, contributes significantly to the global AMR problem.
3. Reduced Drug Accumulation: Preventing Entry
This mechanism involves reducing the concentration of the antibiotic inside the bacterial cell. This can occur through several pathways, including impaired drug uptake or increased drug efflux. It’s like preventing the "key" from even reaching the door.
Examples of Reduced Drug Accumulation:
- Impaired drug uptake: Some bacteria have altered porins, membrane proteins that facilitate antibiotic entry. Changes in porin structure or expression can reduce the amount of antibiotic that enters the cell. This is observed with resistance to several classes of antibiotics, including beta-lactams and aminoglycosides. The alterations in the structure of these proteins impact the drug's permeability across the bacterial membrane.
- Increased drug efflux: Many bacteria possess efflux pumps, membrane proteins that actively transport antibiotics out of the cell. These pumps can remove a wide range of antibiotics, conferring multidrug resistance. Efflux pumps are energy-dependent transmembrane transporters that can actively export a broad range of structurally diverse antimicrobials. Overexpression of these pumps increases their efficiency, leading to high levels of resistance.
Significance: Reduced drug accumulation can lead to significant reductions in intracellular antibiotic concentrations, making it difficult for the drug to reach its target site at therapeutic concentrations. The overexpression or mutation of porins and efflux pumps is a significant contributor to antimicrobial resistance.
4. Target Overproduction/Bypass: Overcoming Inhibition
In this mechanism, the microbe increases the production of its target enzyme or develops an alternative pathway to bypass the drug's action. It is essentially increasing the number of "locks" or creating a new "door" altogether.
Examples of Target Overproduction/Bypass:
- Dihydrofolate reductase (DHFR) overproduction: Trimethoprim inhibits DHFR, an enzyme crucial for folic acid synthesis in bacteria. Bacteria can develop resistance by overproducing DHFR, overcoming the drug's inhibitory effect. This increased production overwhelms the drug's ability to inhibit the enzyme's activity.
- Alternative metabolic pathways: Some bacteria can bypass the drug's target by using alternative metabolic pathways. For instance, resistance to sulfonamides can occur through the uptake of preformed folate from the environment, bypassing the need for the enzyme inhibited by the drug. These alternative pathways circumvent the drug's mechanism of action, leading to reduced susceptibility.
Significance: Target overproduction essentially dilutes the impact of the antibiotic, while bypass mechanisms circumvent the drug's action altogether. Both strategies significantly contribute to antimicrobial resistance.
5. Efflux Pumps: The Cellular Vacuum Cleaners
Efflux pumps are specialized membrane proteins that actively transport various antimicrobial agents out of the bacterial cell. They are an integral part of the reduced drug accumulation mechanism, but their importance warrants separate discussion. They act like cellular vacuum cleaners, constantly removing antibiotics from the cell's interior.
Characteristics of Efflux Pumps:
- Broad substrate specificity: Many efflux pumps can expel a wide range of chemically diverse antibiotics, contributing to multidrug resistance. This broad specificity is a significant concern, as it renders bacteria resistant to multiple classes of antibiotics simultaneously.
- Energy dependence: Efflux pumps require energy to function, often using proton motive force. This ensures continuous removal of antibiotics from the cell. The energy-dependent nature of these pumps ensures the continuous expulsion of antimicrobial agents, even under conditions of high external antibiotic concentrations.
- Regulation: The expression of efflux pumps can be regulated, often increasing in response to antibiotic exposure. This adaptive response increases the bacteria's resistance to antibiotics. The increased expression further exacerbates the resistance mechanism.
Significance: Efflux pumps are a major contributor to multidrug resistance, especially in Gram-negative bacteria, which possess more extensive efflux pump systems than Gram-positive bacteria. The ability of efflux pumps to transport a wide range of antibiotics across the bacterial membrane is a key factor driving the global spread of multidrug resistance.
Conclusion: A Multifaceted Challenge
The five major mechanisms of antimicrobial resistance—target modification, enzymatic inactivation, reduced drug accumulation, target overproduction/bypass, and efflux pumps—are interconnected and often work in concert to confer high levels of resistance. Understanding these mechanisms is crucial for developing effective strategies to combat AMR. These strategies need to focus not only on developing new drugs but also on preventing the spread of resistance genes, optimizing antibiotic use, and exploring alternative therapeutic approaches. The global fight against AMR requires a multipronged approach combining research, policy, and public awareness to effectively tackle this critical threat to human health. The interconnectedness of these mechanisms underscores the complexity of this global health challenge and highlights the need for innovative and comprehensive strategies to combat antimicrobial resistance.
Latest Posts
Latest Posts
-
Unit 2 Algebraic Expressions Answer Key
Apr 04, 2025
-
6 10 6 Handling Multiple Exceptions Vending Machine Example
Apr 04, 2025
-
Select The Most Acidic Type Of Hydrogen In This Molecule
Apr 04, 2025
-
Managerial Accounting Information Is Normally Provided To Managers
Apr 04, 2025
-
What Are Two More Purposes Of The Violent Incident Log
Apr 04, 2025
Related Post
Thank you for visiting our website which covers about Select The Five Major Mechanisms Of Antimicrobial Resistance . We hope the information provided has been useful to you. Feel free to contact us if you have any questions or need further assistance. See you next time and don't miss to bookmark.