Select The Most Acidic Type Of Hydrogen In This Molecule
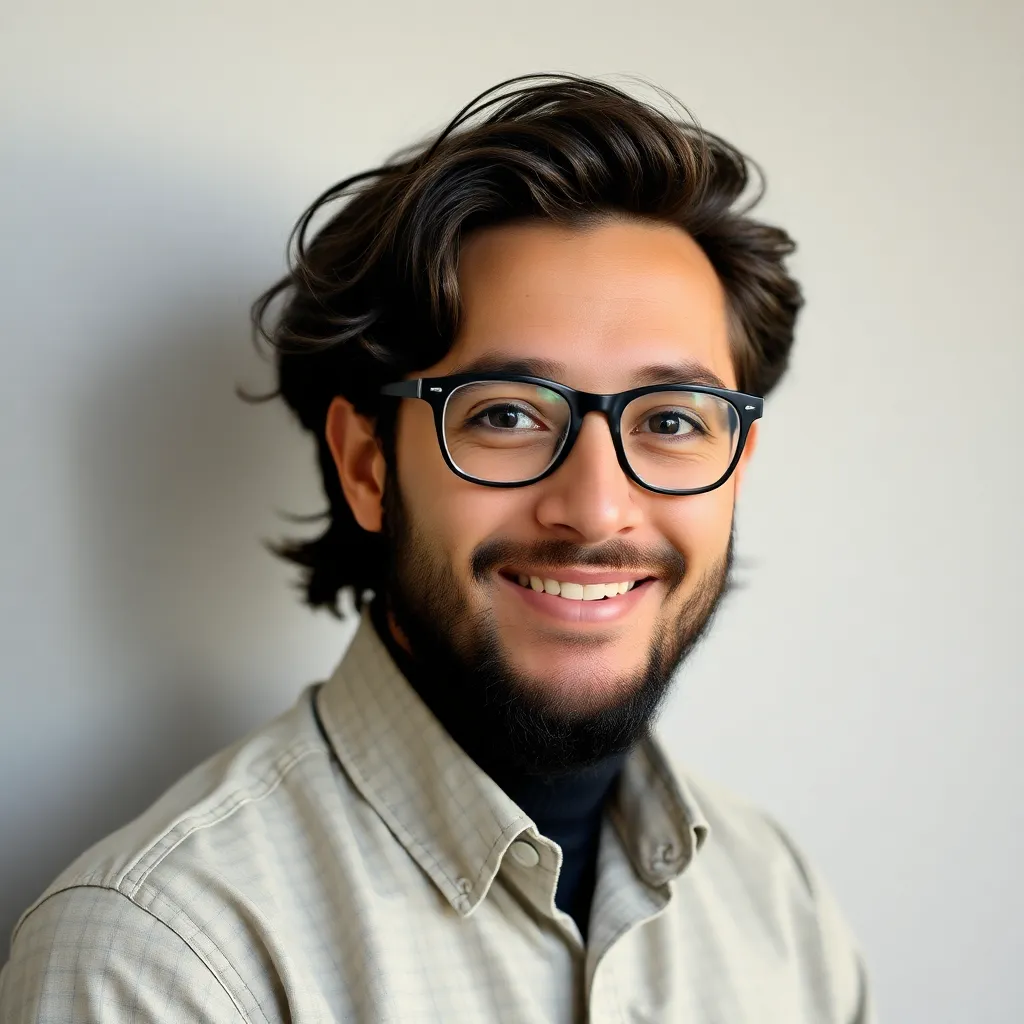
Holbox
Apr 04, 2025 · 6 min read

Table of Contents
- Select The Most Acidic Type Of Hydrogen In This Molecule
- Table of Contents
- Selecting the Most Acidic Hydrogen in a Molecule: A Comprehensive Guide
- Understanding Acidity: A Foundation
- Factors Affecting Conjugate Base Stability and Acidity
- Step-by-Step Approach to Identifying the Most Acidic Hydrogen
- Examples: Illustrating the Principles
- Advanced Considerations and Applications
- Latest Posts
- Latest Posts
- Related Post
Selecting the Most Acidic Hydrogen in a Molecule: A Comprehensive Guide
Determining the most acidic hydrogen in a molecule is a crucial concept in organic chemistry, impacting reactivity, synthesis strategies, and understanding chemical behavior. This involves a careful consideration of several factors, including the stability of the resulting conjugate base, inductive effects, resonance stabilization, and hybridization. This article will provide a comprehensive exploration of these factors and offer a step-by-step approach to identifying the most acidic proton within diverse molecular structures.
Understanding Acidity: A Foundation
Before diving into specific examples, let's establish a clear understanding of acidity. Acidity is a measure of a molecule's tendency to donate a proton (H⁺). The stronger the acid, the more readily it loses a proton. This propensity is quantified by the acid dissociation constant (Ka), with a larger Ka indicating greater acidity. Conversely, the pKa (pKa = -log Ka) is frequently used, where a smaller pKa signifies stronger acidity.
The key to determining acidity lies in understanding the stability of the conjugate base formed after proton loss. The more stable the conjugate base, the stronger the acid. Several factors contribute to conjugate base stability:
Factors Affecting Conjugate Base Stability and Acidity
-
Inductive Effects: Electronegative atoms (like oxygen, nitrogen, fluorine, chlorine, bromine, and iodine) withdraw electron density through sigma bonds. This effect, known as the inductive effect, stabilizes the negative charge on the conjugate base by pulling electron density away from the negatively charged atom. The closer the electronegative atom is to the negatively charged carbon, the stronger the inductive effect and the more stable the conjugate base.
-
Resonance Stabilization: If the negative charge on the conjugate base can be delocalized through resonance, the base becomes significantly more stable. Resonance involves the movement of electrons through pi bonds and lone pairs, distributing the negative charge across multiple atoms. The greater the extent of resonance, the more stable the conjugate base and the stronger the acid.
-
Hybridization: The hybridization of the atom bearing the negative charge influences its stability. The more s-character in the hybrid orbital, the closer the electrons are to the nucleus and the more stable the anion. Therefore, sp hybridized carbons are more electronegative than sp² hybridized carbons, which are, in turn, more electronegative than sp³ hybridized carbons. This translates to sp hybridized carbons forming the most stable conjugate bases and thus being the most acidic.
-
Aromaticity: Aromatic compounds are exceptionally stable due to their delocalized pi electron system. If the conjugate base can achieve aromaticity, it will be significantly stabilized, making the corresponding acid very strong.
-
Size and Steric Effects: Larger atoms can accommodate negative charges more effectively due to their increased surface area. However, steric hindrance can destabilize the conjugate base by creating unfavorable interactions between neighboring atoms or groups.
Step-by-Step Approach to Identifying the Most Acidic Hydrogen
Let's outline a methodical approach to identifying the most acidic proton in a molecule:
-
Identify all potentially acidic hydrogens: These are typically hydrogens bonded to electronegative atoms (O, N, S, halogens) or to carbon atoms adjacent to electronegative atoms.
-
Assess inductive effects: Examine the presence and proximity of electronegative atoms to each potential acidic hydrogen. The closer the electronegative atom, the stronger the inductive effect and the greater the acidity.
-
Evaluate resonance stabilization: Determine if the conjugate base formed after proton removal can be stabilized by resonance. Draw resonance structures to assess the extent of delocalization.
-
Consider hybridization: Identify the hybridization of the atom bearing the negative charge in each possible conjugate base. sp hybridized carbons will form the most stable conjugate base.
-
Check for aromaticity: Determine if the conjugate base can achieve aromaticity. Aromatic conjugate bases are exceptionally stable.
-
Account for steric effects: Consider any steric hindrance that might destabilize the conjugate base.
-
Compare the stability of all possible conjugate bases: Based on the factors above, compare the stability of the conjugate bases formed from each potential acidic hydrogen. The conjugate base with the greatest stability corresponds to the most acidic hydrogen.
Examples: Illustrating the Principles
Let's apply these principles to several example molecules:
Example 1: Acetic Acid (CH₃COOH)
In acetic acid, the hydrogen attached to the oxygen atom (–OH group) is significantly more acidic than the hydrogens attached to the methyl group (–CH₃). The conjugate base, acetate ion (CH₃COO⁻), is resonance-stabilized, distributing the negative charge across both oxygen atoms. This resonance stabilization greatly enhances the stability of the conjugate base, making the –OH hydrogen much more acidic.
Example 2: Phenol (C₆H₅OH)
Phenol exhibits similar behavior to acetic acid. The hydrogen bonded to the oxygen is significantly more acidic than the hydrogens on the aromatic ring. The resulting phenoxide ion is resonance-stabilized by delocalization of the negative charge over the aromatic ring.
Example 3: Comparison of Alcohols and Alkanes
Compare methanol (CH₃OH) and methane (CH₄). The hydrogen of the hydroxyl group in methanol is considerably more acidic than any hydrogen in methane. This difference stems from the resonance stabilization of the methoxide ion (CH₃O⁻) compared to the instability of the hypothetical methyl anion (CH₃⁻). The oxygen atom in the methoxide ion is significantly more electronegative and stabilizes the negative charge.
Example 4: Dicarboxylic Acids
Consider malonic acid (HOOCCH₂COOH). Both carboxylic acid groups are equally acidic because the negative charge on one carboxylate group is inductively stabilized by the second carboxyl group, and vice versa. The first deprotonation is easier than the second due to the inductive effect, thus illustrating a stepwise increase in pKa values.
Example 5: β-Dicarbonyl Compounds
β-dicarbonyl compounds, such as acetylacetone, demonstrate exceptional acidity at the central carbon. This acidity is a result of the remarkable resonance stabilization of the enolate anion, which can distribute the negative charge over two oxygen atoms. This extensive delocalization makes the central hydrogen exceptionally acidic, leading to a pKa significantly lower than that of typical ketones or aldehydes.
Example 6: Influence of Electronegative Substituents
Consider trifluoroacetic acid (CF₃COOH) versus acetic acid (CH₃COOH). The presence of three highly electronegative fluorine atoms in trifluoroacetic acid exerts a powerful inductive effect. This effect dramatically stabilizes the trifluoroacetate anion, making trifluoroacetic acid a significantly stronger acid than acetic acid.
Advanced Considerations and Applications
The principles discussed above form the basis for understanding acidity in organic molecules. However, more advanced aspects, such as the Hammett equation (quantifying substituent effects), the influence of solvent effects, and the use of computational methods (like DFT calculations) can further refine the prediction of acidity. These advanced concepts are crucial for understanding complex chemical systems and designing new reactions and materials. These tools allow for a more precise estimation of pKa values and can consider the nuanced interplay of factors that influence acidity.
The ability to identify the most acidic hydrogen is a vital skill in organic chemistry. It is central to predicting reaction outcomes, designing synthetic pathways, and comprehending the behavior of diverse organic molecules. The principles of inductive effects, resonance stabilization, hybridization, and aromaticity are fundamental tools for this crucial task. By systematically analyzing these factors, one can effectively determine the most acidic hydrogen in a given molecule, providing a cornerstone for a deep understanding of organic reactivity.
Latest Posts
Latest Posts
-
With Parent Company The Simulation Means
Apr 09, 2025
-
The Stream Function For An Incompressible Two Dimensional Flow Field Is
Apr 09, 2025
-
Determine The Hybridization And Geometry Around The Indicated Carbon Atoms
Apr 09, 2025
-
The Phrase Behavioral Expressions Of Distress Refers To
Apr 09, 2025
-
The Ph Scale Is A Mathematical Indicator Of
Apr 09, 2025
Related Post
Thank you for visiting our website which covers about Select The Most Acidic Type Of Hydrogen In This Molecule . We hope the information provided has been useful to you. Feel free to contact us if you have any questions or need further assistance. See you next time and don't miss to bookmark.