Construct A Simulated Proton-decoupled 13c Nmr
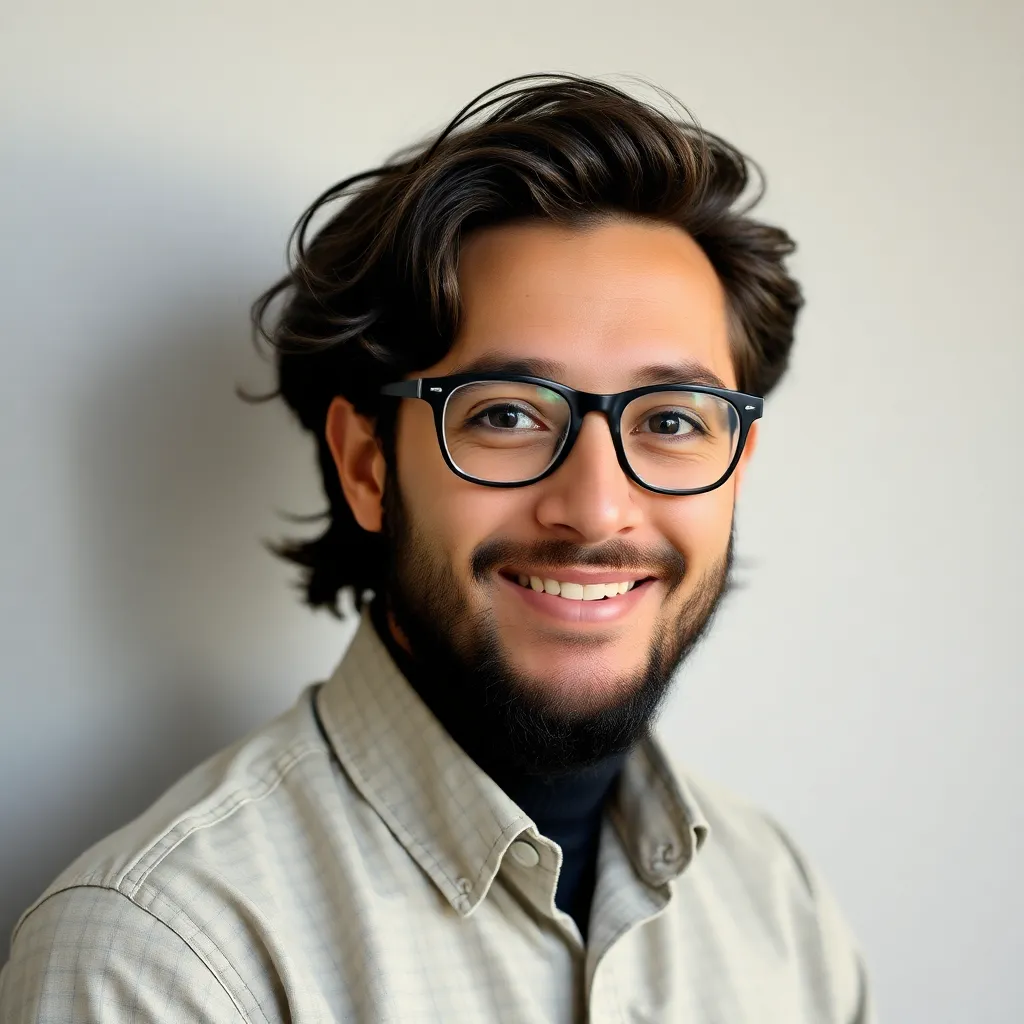
Holbox
Mar 24, 2025 · 6 min read
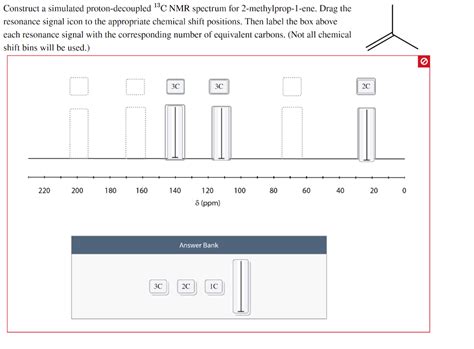
Table of Contents
- Construct A Simulated Proton-decoupled 13c Nmr
- Table of Contents
- Constructing a Simulated Proton-Decoupled ¹³C NMR Spectrum
- Understanding Proton-Decoupled ¹³C NMR
- Steps in Simulating a Proton-Decoupled ¹³C NMR Spectrum
- 1. Defining the Molecular Structure
- 2. Assigning Chemical Shifts
- 3. Considering Coupling Constants (Before Decoupling)
- 4. Implementing Proton Decoupling
- 5. Spectral Representation and Interpretation
- Example: Simulating the Proton-Decoupled ¹³C NMR of Ethanol (CH₃CH₂OH)
- Advanced Considerations
- Conclusion
- Latest Posts
- Latest Posts
- Related Post
Constructing a Simulated Proton-Decoupled ¹³C NMR Spectrum
Nuclear Magnetic Resonance (NMR) spectroscopy is a powerful analytical technique used extensively in chemistry and related fields to determine the structure and properties of molecules. Specifically, ¹³C NMR spectroscopy provides invaluable information about the carbon atoms within a molecule. However, ¹³C NMR spectra are often complicated by the coupling of ¹³C nuclei to neighboring protons, leading to complex multiplet patterns. To simplify these spectra and focus on chemical shifts, proton decoupling is frequently employed. This article will guide you through the process of constructing a simulated proton-decoupled ¹³C NMR spectrum, covering the underlying principles, the necessary steps, and the interpretation of the results. While we won't be using any specific software, the principles outlined here are applicable to various NMR simulation packages.
Understanding Proton-Decoupled ¹³C NMR
Before diving into the simulation, let's review the fundamentals of proton-decoupled ¹³C NMR. In a standard ¹³C NMR experiment, each carbon atom interacts with its neighboring protons, resulting in spin-spin coupling. This coupling manifests as multiplets in the spectrum – a singlet for a carbon with no directly attached protons, a doublet for a carbon with one directly attached proton, a triplet for a carbon with two directly attached protons, and so on, following the n+1 rule (where n is the number of equivalent neighboring protons). These multiplets often overlap, making spectral interpretation challenging.
Proton decoupling simplifies the spectrum dramatically. By irradiating the sample with a strong radiofrequency field at the proton resonance frequency, the ¹³C nuclei effectively "see" an average of the proton spin states. This collapses all the multiplets into single peaks, corresponding to the individual carbon atoms' chemical shifts. The resulting spectrum is much cleaner, with each peak representing a unique carbon environment.
Steps in Simulating a Proton-Decoupled ¹³C NMR Spectrum
Simulating a proton-decoupled ¹³C NMR spectrum requires several key steps:
1. Defining the Molecular Structure
The first and most critical step is precisely defining the molecular structure of the compound you want to simulate. This involves specifying the connectivity of all atoms (carbon and hydrogen) and their three-dimensional arrangement. This information is crucial because the chemical shift of a carbon atom is highly dependent on its immediate chemical environment – the types of atoms bonded to it, the presence of electron-withdrawing or electron-donating groups, and the overall molecular geometry. Accurate input is paramount for a realistic simulation.
2. Assigning Chemical Shifts
The next step involves assigning chemical shifts (δ) to each carbon atom in the molecule. Chemical shifts are expressed in parts per million (ppm) relative to a standard (usually tetramethylsilane, TMS). Predicting chemical shifts accurately requires a good understanding of NMR principles and the effects of different substituents. There are several resources available to assist in this process:
- Empirical correlations: Extensive tables and databases exist that correlate the chemical shifts of carbon atoms with their structural environment. These correlations are often based on experimental data from a large number of compounds.
- Computational chemistry: Sophisticated computational methods can predict chemical shifts with high accuracy. These methods often require specialized software and computational resources but offer excellent results.
It's important to remember that predicted chemical shifts are only approximations; small discrepancies between predicted and experimental values are common.
3. Considering Coupling Constants (Before Decoupling)
Before implementing proton decoupling, consider the coupling constants (J) between the ¹³C and ¹H nuclei. Coupling constants represent the strength of the interaction between the two nuclei and determine the splitting patterns (multiplets) in the ¹³C NMR spectrum. They are typically expressed in Hertz (Hz). The magnitude of the coupling constant is influenced by factors such as the number of bonds separating the coupled nuclei, the hybridization of the carbon atom, and the nature of the intervening bonds. This step is crucial for generating a realistic coupled spectrum before applying the decoupling. This allows for a direct comparison of coupled and decoupled spectra, highlighting the effect of decoupling.
4. Implementing Proton Decoupling
This is where the simulation's magic happens. To simulate proton decoupling, you essentially collapse all multiplets into singlets, retaining only the chemical shift information. In a simulation, this is implemented algorithmically by averaging the chemical shifts across all coupled states. Imagine the simulated spectrum as being overlaid with the various multiplets; after decoupling, only the central chemical shift of each multiplet remains, resulting in a considerably simplified spectrum. This is the key distinction between a standard ¹³C NMR simulation and a proton-decoupled one.
5. Spectral Representation and Interpretation
The final step involves representing the simulated spectrum graphically. This will typically consist of a plot with chemical shift (ppm) on the x-axis and intensity (arbitrary units) on the y-axis. Each carbon atom will be represented by a single peak at its predicted chemical shift. The height of the peak might reflect the number of equivalent carbon atoms in the molecule (though this might not always be the case depending on your simulation parameters), assisting in the integration of the signal. Accurate peak assignment is then crucial for properly interpreting the resulting spectrum.
Example: Simulating the Proton-Decoupled ¹³C NMR of Ethanol (CH₃CH₂OH)
Let's walk through a simplified example using ethanol (CH₃CH₂OH).
-
Molecular Structure: Ethanol has three carbon atoms: one methyl (CH₃), one methylene (CH₂), and one carbonyl carbon (C) of the hydroxyl group.
-
Chemical Shift Assignment: Based on typical chemical shift ranges:
- Methyl (CH₃): ~15-20 ppm
- Methylene (CH₂): ~30-40 ppm
- Carbonyl carbon (C): ~50-70 ppm (this range is shifted because of the attached oxygen). The exact value would depend on solvent and other factors.
-
Coupling Constants (Before Decoupling): We would expect to see coupling between the ¹³C and ¹H nuclei in the methyl and methylene groups. These couplings would produce multiplets. The magnitude of J would depend on the specific environment.
-
Proton Decoupling: Applying proton decoupling would collapse the multiplets from the methyl and methylene carbons into single peaks at their respective chemical shifts.
-
Spectral Representation: The simulated proton-decoupled ¹³C NMR spectrum would show three distinct peaks, approximately at 15-20 ppm, 30-40 ppm, and 50-70 ppm, representing the methyl, methylene, and carbonyl carbon atoms respectively. The integration would approximately reflect the 1:2:1 ratio of the different carbon types.
Advanced Considerations
While this example simplifies the process, several advanced considerations might affect the accuracy and complexity of the simulation:
-
Solvent effects: The solvent used in an NMR experiment can significantly influence the chemical shifts of the carbon atoms. The simulation should ideally account for these effects.
-
Temperature effects: Temperature can also slightly shift chemical shifts.
-
Dynamic processes: If the molecule undergoes any dynamic processes, like rotation or conformational changes, this can affect the appearance of the spectrum.
-
Relaxation times: Relaxation times (T₁ and T₂) influence peak intensities and line widths in an actual NMR spectrum. A complete simulation would account for these effects.
Conclusion
Simulating a proton-decoupled ¹³C NMR spectrum is a valuable tool for understanding the relationship between molecular structure and NMR data. While the process might seem complex, understanding the underlying principles of chemical shifts, coupling constants, and proton decoupling is paramount. This allows for a deeper appreciation of the power of NMR spectroscopy in structural elucidation and provides a solid foundation for interpreting experimental spectra effectively. Although we haven't delved into specific software applications, the fundamental steps outlined here remain consistent across various simulation programs, empowering you to construct accurate and insightful simulations. Remember that the accuracy of the simulation critically hinges on the precision of input parameters, particularly chemical shift assignments and molecular structure definition.
Latest Posts
Latest Posts
-
Three Of The Four Types Of Processes Are
Mar 29, 2025
-
Reference Groups Have One Of The Following Implications
Mar 29, 2025
-
You Manage A Farm Equipment Supply Store
Mar 29, 2025
-
Alan Is Recording Payroll That Was Processed Outside Of Quickbooks
Mar 29, 2025
-
A Firms Cash Flow From Investing Activities Includes
Mar 29, 2025
Related Post
Thank you for visiting our website which covers about Construct A Simulated Proton-decoupled 13c Nmr . We hope the information provided has been useful to you. Feel free to contact us if you have any questions or need further assistance. See you next time and don't miss to bookmark.