Which Compound Does Not Have A Linear Molecular Geometry
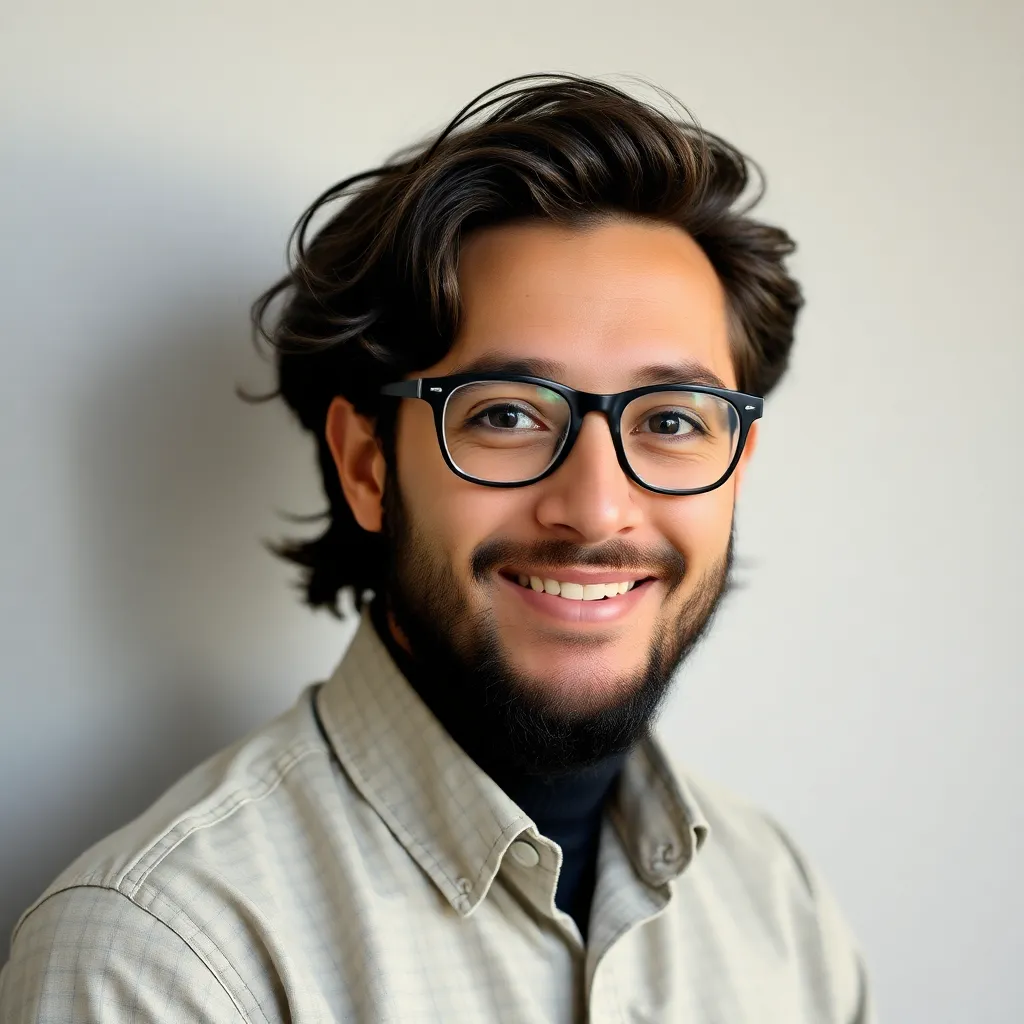
Holbox
Mar 30, 2025 · 6 min read

Table of Contents
- Which Compound Does Not Have A Linear Molecular Geometry
- Table of Contents
- Which Compound Does Not Have a Linear Molecular Geometry?
- Understanding Molecular Geometry: VSEPR Theory
- Lone Pairs: The Geometry Benders
- Multiple Bonds: The Space Occupiers
- Examples of Compounds with Non-Linear Molecular Geometries
- 1. Water (H₂O): Bent Geometry
- 2. Ammonia (NH₃): Trigonal Pyramidal Geometry
- 3. Sulfur Dioxide (SO₂): Bent Geometry
- 4. Methane (CH₄): Tetrahedral Geometry
- 5. Carbon Dioxide (CO₂): A Linear Exception
- Factors Influencing Molecular Geometry Beyond VSEPR
- Conclusion: The Diverse World of Molecular Shapes
- Latest Posts
- Latest Posts
- Related Post
Which Compound Does Not Have a Linear Molecular Geometry?
Understanding molecular geometry is crucial in chemistry, as it dictates a molecule's properties, including reactivity, polarity, and physical state. Linear geometry, characterized by a 180° bond angle, is just one of several possible arrangements. Many compounds exhibit this simple structure, but a significant number deviate, adopting more complex shapes. This article delves into the intricacies of molecular geometry, exploring various factors that influence it and focusing specifically on compounds that do not possess a linear molecular geometry.
Understanding Molecular Geometry: VSEPR Theory
The Valence Shell Electron Pair Repulsion (VSEPR) theory is a cornerstone in predicting molecular geometry. This theory posits that electron pairs—both bonding and non-bonding (lone pairs)—repel each other, arranging themselves to maximize distance and minimize repulsion. This arrangement dictates the overall shape of the molecule. The key to understanding non-linear geometries lies in considering the influence of lone pairs and multiple bonds.
Lone Pairs: The Geometry Benders
Lone pairs of electrons occupy more space than bonding pairs. This increased spatial demand causes a distortion in the bond angles, pushing bonding pairs closer together and resulting in a deviation from linearity. The presence of even a single lone pair significantly alters the molecular geometry.
Multiple Bonds: The Space Occupiers
Double and triple bonds also occupy more space than single bonds. While they don't cause as significant a distortion as lone pairs, they still influence the bond angles and can contribute to non-linear geometries, especially in combination with lone pairs.
Examples of Compounds with Non-Linear Molecular Geometries
Let's explore several examples of compounds that deviate from linear geometry, highlighting the influence of lone pairs and multiple bonds:
1. Water (H₂O): Bent Geometry
Water is a classic example of a molecule with a bent geometry. The oxygen atom is centrally located, bonded to two hydrogen atoms. However, oxygen also possesses two lone pairs of electrons. These lone pairs exert a stronger repulsive force than the bonding pairs, compressing the H-O-H bond angle to approximately 104.5°, significantly less than the 180° expected in a linear molecule. This bent shape contributes to water's high polarity and exceptional solvent properties.
Key takeaway: The presence of two lone pairs on the central oxygen atom is the primary reason why water does not have a linear molecular geometry.
2. Ammonia (NH₃): Trigonal Pyramidal Geometry
Ammonia features a nitrogen atom at the center, bonded to three hydrogen atoms and possessing one lone pair of electrons. The lone pair's repulsive force pushes the three hydrogen atoms closer together, resulting in a trigonal pyramidal shape. The bond angles are approximately 107°, again deviating considerably from the 180° of a linear structure. The presence of this lone pair is pivotal in determining ammonia's properties, including its basicity.
Key takeaway: The single lone pair on the central nitrogen atom is responsible for the non-linear trigonal pyramidal geometry of ammonia.
3. Sulfur Dioxide (SO₂): Bent Geometry
Sulfur dioxide presents a slightly more complex scenario. The sulfur atom is double-bonded to one oxygen atom and single-bonded to another. Additionally, sulfur possesses one lone pair of electrons. The combination of a double bond and a lone pair distorts the molecule, giving it a bent shape with a bond angle less than 120°. This bent structure contributes to sulfur dioxide's polar nature and its role as a potent air pollutant.
Key takeaway: The combination of a double bond and a lone pair on the central sulfur atom prevents a linear geometry, resulting in a bent molecule.
4. Methane (CH₄): Tetrahedral Geometry
Methane, while not linear, serves as a crucial comparison point. The central carbon atom is surrounded by four hydrogen atoms, forming a tetrahedral structure with bond angles of approximately 109.5°. While not linear, it's essential to note that the absence of lone pairs on the carbon atom results in a symmetrical, albeit non-linear, geometry. This symmetry minimizes the dipole moment, making methane a non-polar molecule.
Key takeaway: The absence of lone pairs and the symmetrical arrangement of four bonding pairs contribute to methane's tetrahedral geometry – a non-linear structure, but different from the bent geometries seen with lone pairs.
5. Carbon Dioxide (CO₂): A Linear Exception
It's crucial to also mention carbon dioxide (CO₂). Unlike the previous examples, CO₂ is linear. The central carbon atom forms double bonds with two oxygen atoms. The symmetrical arrangement of these double bonds, with no lone pairs on the central atom, results in a linear molecule with a bond angle of 180°. This linear structure contributes to its non-polar nature. This serves as a key example to highlight the importance of both the number of electron domains and their distribution around the central atom.
Key takeaway: The symmetrical arrangement of two double bonds around the central carbon atom, in the absence of lone pairs, leads to the linear molecular geometry of CO2. This contrasts with the examples above, showcasing the role of lone pairs and unequal bond distributions in generating non-linear structures.
Factors Influencing Molecular Geometry Beyond VSEPR
While VSEPR theory is highly effective in predicting molecular geometry, it's not the only influencing factor. Other factors include:
-
Hybridization: The hybridization of atomic orbitals significantly impacts bond angles and molecular shape. Different hybridization states (sp, sp², sp³) lead to different geometries.
-
Steric effects: The size and bulkiness of atoms or groups can influence bond angles and overall molecular shape through steric hindrance.
-
Resonance: In molecules exhibiting resonance, the delocalization of electrons can affect bond lengths and angles, influencing the final molecular geometry.
-
Hydrogen bonding: Hydrogen bonding can influence the overall arrangement of molecules in a substance, affecting macroscopic properties even if it doesn't directly alter the geometry of an individual molecule.
Conclusion: The Diverse World of Molecular Shapes
Numerous compounds deviate from the simple linear geometry. The presence of lone pairs and multiple bonds, along with factors such as hybridization and steric effects, significantly contribute to the diverse range of molecular shapes observed in nature. Understanding these factors is crucial in predicting and explaining the physical and chemical properties of molecules. By applying VSEPR theory and considering other influencing factors, we can accurately predict and comprehend the intricate world of molecular geometries, moving beyond the simplified picture of linear molecules to appreciate the complexities of three-dimensional structures. The examples discussed here showcase how different combinations of bonding and non-bonding electron pairs contribute to a diverse range of molecular structures, highlighting the importance of these concepts in chemistry. Further exploration of these principles provides a deeper understanding of the relationships between molecular structure and properties.
Latest Posts
Latest Posts
-
Match The Hormone Abbreviations With Their Function
Apr 02, 2025
-
What Is Are The Product S Of The Following Reaction
Apr 02, 2025
-
Not Yielding To Visually Impaired Pedestrians Is A Misdemeanor
Apr 02, 2025
-
Asking About A Persons Place Of Origin
Apr 02, 2025
-
Section 3 Graded Questions Understanding Experimental Design
Apr 02, 2025
Related Post
Thank you for visiting our website which covers about Which Compound Does Not Have A Linear Molecular Geometry . We hope the information provided has been useful to you. Feel free to contact us if you have any questions or need further assistance. See you next time and don't miss to bookmark.