Saltatory Conduction Is Made Possible By
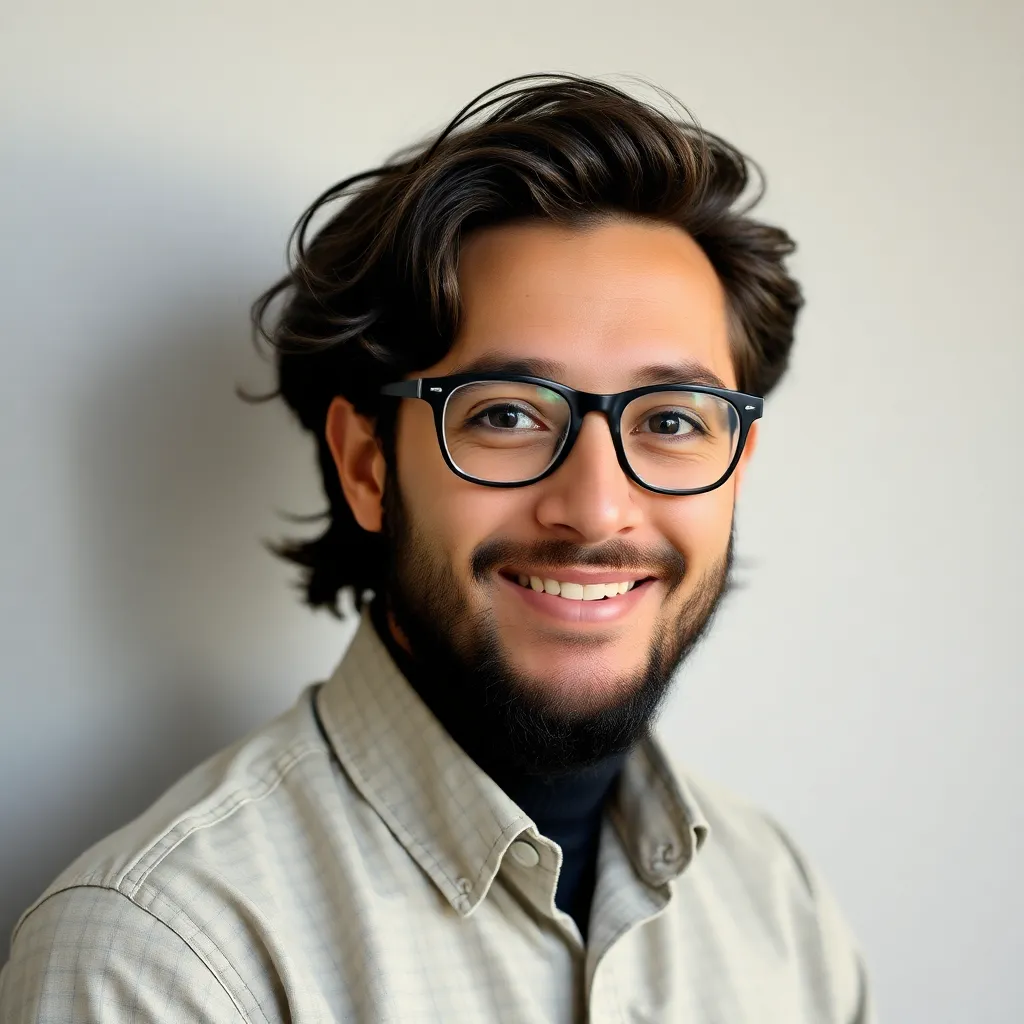
Holbox
Apr 02, 2025 · 7 min read

Table of Contents
- Saltatory Conduction Is Made Possible By
- Table of Contents
- Saltatory Conduction: The Myelin Sheath's Crucial Role in Speedy Nerve Impulse Transmission
- Understanding the Myelin Sheath: The Foundation of Saltatory Conduction
- The Role of Oligodendrocytes and Schwann Cells
- The Mechanism of Saltatory Conduction: A Detailed Explanation
- Depolarization at the Nodes of Ranvier
- Passive Current Flow and Action Potential Regeneration
- The Importance of Node of Ranvier Density and Myelin Thickness
- Comparison with Continuous Conduction: Speed and Efficiency
- Diseases Affecting Saltatory Conduction: Demyelinating Diseases
- Multiple Sclerosis (MS)
- Guillain-Barré Syndrome (GBS)
- Charcot-Marie-Tooth Disease (CMT)
- The Future of Research: Understanding and Treating Myelin Disorders
- Conclusion: The Essential Role of Myelin in Neural Function
- Latest Posts
- Latest Posts
- Related Post
Saltatory Conduction: The Myelin Sheath's Crucial Role in Speedy Nerve Impulse Transmission
Saltatory conduction is a fascinating process that significantly speeds up the transmission of nerve impulses along axons. Unlike continuous conduction, where the impulse travels smoothly along the axon membrane, saltatory conduction involves the impulse "jumping" between specific points along the axon. This "leapfrogging" action dramatically increases the speed of signal transmission, enabling rapid responses to stimuli and efficient communication within the nervous system. But what makes saltatory conduction possible? The answer lies in a specialized structure surrounding many axons: the myelin sheath.
Understanding the Myelin Sheath: The Foundation of Saltatory Conduction
The myelin sheath is a fatty insulating layer that wraps around the axons of many neurons. It's not a continuous covering, but rather segmented, with gaps known as Nodes of Ranvier occurring between the myelin segments. These nodes are crucial for the mechanism of saltatory conduction. The myelin sheath itself is formed by specialized glial cells: oligodendrocytes in the central nervous system (CNS) and Schwann cells in the peripheral nervous system (PNS). These cells wrap their membranes repeatedly around the axon, forming the concentric layers of myelin.
The Role of Oligodendrocytes and Schwann Cells
Both oligodendrocytes and Schwann cells play an essential role in creating and maintaining the myelin sheath. Oligodendrocytes, found in the brain and spinal cord, can myelinate multiple axons simultaneously, extending their processes to wrap around several axons. Schwann cells, on the other hand, found in the peripheral nerves, myelinate only a single axon segment. The precise wrapping process and the formation of the myelin sheath are complex, involving intricate cell-cell interactions and signaling pathways. Dysfunctions in these processes can lead to demyelinating diseases, such as multiple sclerosis.
The Mechanism of Saltatory Conduction: A Detailed Explanation
Saltatory conduction relies on the unique structure of the myelinated axon. The myelin sheath acts as an insulator, preventing ion flow across the axon membrane in the myelinated segments (internodes). This insulation dramatically increases the membrane's resistance to ion movement, forcing the action potential to jump from one Node of Ranvier to the next.
Depolarization at the Nodes of Ranvier
The process begins with the depolarization of the axon membrane at the initial segment of the axon (axon hillock). This depolarization triggers an action potential, which propagates down the axon. However, due to the myelin insulation, the action potential doesn't travel continuously along the axon membrane. Instead, the current flows passively along the internode, under the myelin sheath, to the next Node of Ranvier.
Passive Current Flow and Action Potential Regeneration
As the current flows passively under the myelin sheath, there is some loss of signal strength due to the resistance of the axon's cytoplasm. However, this passive spread is sufficient to reach the next Node of Ranvier with enough strength to trigger the opening of voltage-gated ion channels. At the Node of Ranvier, a high density of voltage-gated sodium channels ensures that the membrane potential reaches threshold. This depolarization regenerates the action potential, effectively boosting the signal.
The Importance of Node of Ranvier Density and Myelin Thickness
The density of Nodes of Ranvier and the thickness of the myelin sheath are crucial factors that influence the speed of saltatory conduction. A higher density of Nodes of Ranvier results in shorter internodes, requiring fewer jumps for the action potential to reach its destination, leading to faster transmission. Similarly, a thicker myelin sheath provides better insulation, reducing the passive current loss along the internode and thereby increasing the speed of conduction. The interplay between these two factors determines the overall efficiency of saltatory conduction.
Comparison with Continuous Conduction: Speed and Efficiency
Continuous conduction, which occurs in unmyelinated axons, involves the sequential depolarization of adjacent membrane segments. The action potential spreads along the entire axon membrane, requiring the sequential opening and closing of ion channels at each point. This process is inherently slower than saltatory conduction because each segment needs to be depolarized individually.
The difference in speed is substantial. Saltatory conduction can be up to 100 times faster than continuous conduction. This speed advantage is crucial for rapid reflexes, coordinated movements, and efficient information processing within the nervous system. The energy efficiency is also markedly higher in saltatory conduction, as fewer ion channels need to be activated along the axon's length, reducing the energy expenditure needed for the nerve impulse to travel.
Diseases Affecting Saltatory Conduction: Demyelinating Diseases
Several diseases impair the myelin sheath, directly affecting the efficiency of saltatory conduction. These are broadly classified as demyelinating diseases, and their impact can range from mild sensory disturbances to severe paralysis.
Multiple Sclerosis (MS)
Multiple sclerosis (MS) is a chronic autoimmune disease affecting the CNS. In MS, the immune system mistakenly attacks and damages the myelin sheath around axons in the brain and spinal cord. This damage disrupts saltatory conduction, leading to a range of neurological symptoms, including muscle weakness, fatigue, vision problems, and balance issues. The unpredictable nature of MS-related demyelination means symptoms can fluctuate significantly.
Guillain-Barré Syndrome (GBS)
Guillain-Barré syndrome (GBS) is a rare autoimmune disorder affecting the PNS. Similar to MS, GBS involves immune system attack on the myelin sheath, but in this case, the damage targets the myelin around peripheral nerves. This leads to muscle weakness and paralysis, typically starting in the legs and spreading upward. While often severe, GBS is usually temporary, with most patients experiencing significant recovery.
Charcot-Marie-Tooth Disease (CMT)
Charcot-Marie-Tooth disease (CMT) is a group of inherited disorders affecting the peripheral nerves. In CMT, genetic mutations cause defects in the myelin sheath or the axons themselves, leading to progressive muscle weakness, atrophy, and sensory loss. The severity and progression of CMT vary greatly depending on the specific genetic mutation involved.
These diseases highlight the critical role of the myelin sheath in maintaining the integrity and speed of nerve impulse transmission. Damage to the myelin sheath directly impacts saltatory conduction, leading to impaired neurological function and various clinical manifestations.
The Future of Research: Understanding and Treating Myelin Disorders
Research into the mechanisms of myelin formation, maintenance, and repair is ongoing. Scientists are exploring novel strategies to promote myelin regeneration and repair in demyelinating diseases. These include:
- Stem cell therapy: Using stem cells to replace damaged myelin-producing cells.
- Immunomodulatory therapies: Suppressing the immune system's attack on myelin.
- Neurotrophic factors: Stimulating the growth and repair of myelin and axons.
Advancements in these areas hold significant promise for improving the lives of individuals affected by demyelinating diseases. A deeper understanding of the molecular mechanisms underlying myelin formation and repair is essential for developing effective therapies that target the root causes of these debilitating conditions. Furthermore, research is exploring potential links between myelin health and other neurological conditions, such as Alzheimer's disease and cognitive decline, suggesting that preserving myelin integrity might play a broader role in maintaining overall brain health.
Conclusion: The Essential Role of Myelin in Neural Function
Saltatory conduction is a remarkable adaptation in the nervous system that allows for rapid and efficient transmission of nerve impulses. The myelin sheath, produced by specialized glial cells, is the key player in this process, enabling the action potential to "jump" between Nodes of Ranvier and dramatically increasing transmission speed. Understanding the intricate mechanism of saltatory conduction and the impact of myelin disorders provides invaluable insights into neurological function and disease pathogenesis. Continued research promises to unveil further secrets of this vital process and lead to innovative treatments for diseases that disrupt the integrity of the myelin sheath. This research is essential not only for treating existing conditions but also for preventing future neurological disorders linked to compromised myelin health. The future of neurology rests, in part, on our continued exploration of this fascinating and crucial aspect of nervous system function.
Latest Posts
Latest Posts
-
Imagine You Have Some Workers And Some Handheld Computers
Apr 04, 2025
-
Post The Transactions To The T Accounts
Apr 04, 2025
-
The Brs Corporation Makes Collections On Sales
Apr 04, 2025
-
Ensuring Data Privacy Is An Essential Skill For Cybersecurity Professionals
Apr 04, 2025
-
Outstanding Common Stock Refers To The Total Number Of Shares
Apr 04, 2025
Related Post
Thank you for visiting our website which covers about Saltatory Conduction Is Made Possible By . We hope the information provided has been useful to you. Feel free to contact us if you have any questions or need further assistance. See you next time and don't miss to bookmark.