How Can A Biological Pathway Contain A Thermodynamically Unfavorable Reaction
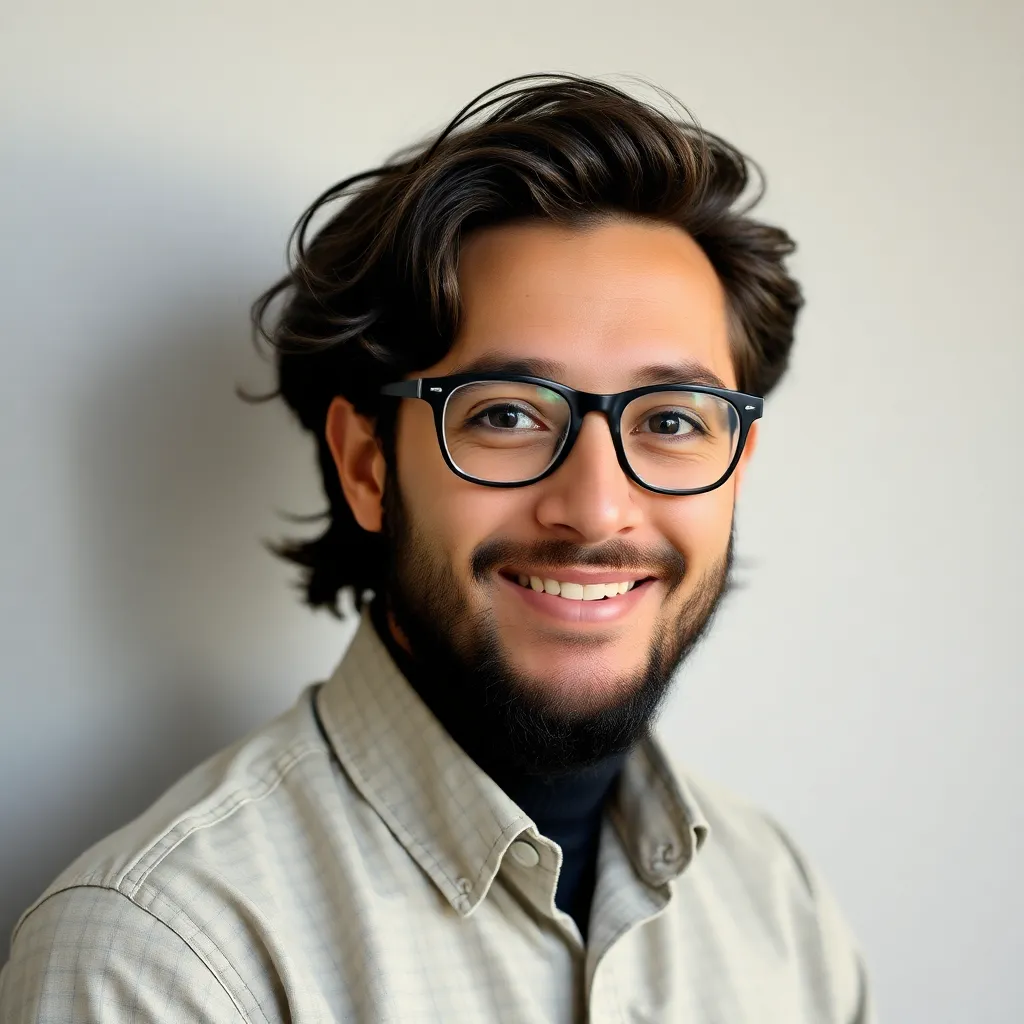
Holbox
Mar 30, 2025 · 5 min read
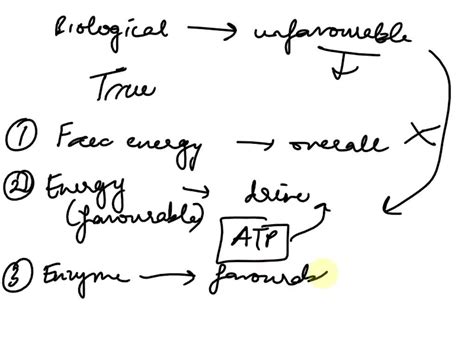
Table of Contents
- How Can A Biological Pathway Contain A Thermodynamically Unfavorable Reaction
- Table of Contents
- How Can a Biological Pathway Contain a Thermodynamically Unfavorable Reaction?
- The Importance of Gibbs Free Energy (ΔG)
- Coupling Unfavorable Reactions with Favorable Ones
- ATP Hydrolysis: The Universal Energy Currency
- Maintaining a High Energy Charge
- The Role of Enzymes
- Compartmentalization: Separating Reactants and Products
- Redox Reactions and Electron Carriers
- Membrane Potential and Ion Gradients
- Environmental Factors
- Conclusion
- Latest Posts
- Latest Posts
- Related Post
How Can a Biological Pathway Contain a Thermodynamically Unfavorable Reaction?
Biological systems are incredibly complex and efficient machines, orchestrating a vast array of chemical reactions to maintain life. These reactions, organized into intricate metabolic pathways, are governed by the principles of thermodynamics. A key challenge arises when considering that some individual reactions within these pathways are thermodynamically unfavorable, meaning they have a positive Gibbs free energy change (ΔG > 0) and would not proceed spontaneously under standard conditions. So, how do these seemingly impossible reactions occur and contribute to the overall functioning of a biological pathway? The answer lies in the ingenious coupling of reactions and the cellular environment's clever manipulation of thermodynamic parameters.
The Importance of Gibbs Free Energy (ΔG)
Before delving into the mechanisms, let's briefly revisit the concept of Gibbs free energy. ΔG represents the change in free energy during a reaction. A negative ΔG indicates a spontaneous reaction that releases energy (exergonic), while a positive ΔG signifies a non-spontaneous reaction requiring energy input (endergonic). In biological systems, the term "favorable" usually refers to exergonic reactions (ΔG < 0), and "unfavorable" to endergonic reactions (ΔG > 0).
Coupling Unfavorable Reactions with Favorable Ones
The most common strategy cells employ to drive thermodynamically unfavorable reactions is coupling them with highly favorable reactions. This essentially means linking an endergonic reaction (ΔG > 0) with an exergonic reaction (ΔG < 0) such that the overall free energy change of the coupled reaction is negative. The energy released by the exergonic reaction provides the necessary energy input for the endergonic reaction to proceed.
A classic example is the synthesis of ATP (adenosine triphosphate), the cell's primary energy currency. The direct synthesis of ATP from ADP (adenosine diphosphate) and inorganic phosphate (Pi) is thermodynamically unfavorable:
ADP + Pi → ATP + H₂O (ΔG > 0)
However, this reaction is frequently coupled with the highly exergonic hydrolysis of other molecules, such as glucose during cellular respiration or phosphoenolpyruvate (PEP) in glycolysis. The energy released during the breakdown of these molecules is harnessed to drive ATP synthesis. The overall coupled reaction becomes exergonic, allowing ATP formation.
ATP Hydrolysis: The Universal Energy Currency
The hydrolysis of ATP plays a central role in numerous coupled reactions. The large negative ΔG of ATP hydrolysis (-30.5 kJ/mol under standard conditions) makes it an excellent energy source to drive otherwise unfavorable reactions. This is achieved through the formation of high-energy phosphorylated intermediates.
ATP + H₂O → ADP + Pi + energy
The phosphate group from ATP is transferred to a reactant molecule, forming a high-energy phosphorylated intermediate. This intermediate then undergoes further reactions, driving the overall pathway forward. The energy released during the subsequent breakdown of the phosphorylated intermediate is used to perform work.
Maintaining a High Energy Charge
The cell meticulously maintains a high energy charge – the ratio of ATP to ADP plus AMP (adenosine monophosphate). A high energy charge favors the reverse reaction, meaning ATP synthesis. This strategic control over the concentration of reactants and products influences the actual ΔG of the reaction and can effectively drive an otherwise unfavorable reaction in the desired direction.
Changes in concentrations of reactants and products can shift the equilibrium and drive reactions towards completion. This principle applies to many metabolic pathways, where enzyme activity regulates the concentrations of intermediates, influencing the direction of reactions even if they are individually unfavorable under standard conditions.
The Role of Enzymes
Enzymes are biological catalysts crucial to life. They don't alter the thermodynamics of a reaction (i.e., they don't change ΔG), but they significantly lower the activation energy (Ea). Activation energy is the energy barrier that must be overcome for a reaction to proceed. By lowering Ea, enzymes accelerate the rate of reaction, allowing thermodynamically favorable reactions to occur at a biologically relevant speed.
Even for thermodynamically unfavorable reactions, enzymes are essential for driving them forward. They increase the rate of the coupled reaction, allowing the favorable process to overcome the energy barrier of the unfavorable one more quickly and efficiently.
Compartmentalization: Separating Reactants and Products
Cellular compartmentalization plays a significant role in thermodynamic manipulation. By physically separating reactants and products within different cellular organelles (e.g., mitochondria, chloroplasts, endoplasmic reticulum), cells can control the local concentrations of these molecules. This helps to maintain a favorable concentration gradient that drives reactions forward, even those that are unfavorable under standard conditions. For instance, the proton gradient across the mitochondrial inner membrane is essential for ATP synthesis in oxidative phosphorylation.
Redox Reactions and Electron Carriers
Many metabolic pathways involve redox reactions (reduction-oxidation), where electrons are transferred between molecules. These reactions can be coupled to drive thermodynamically unfavorable processes. Electron carriers, such as NADH and FADH₂, play a vital role in these coupled reactions. They accept electrons from exergonic redox reactions and donate them to endergonic redox reactions, mediating the energy transfer and driving the unfavorable process. The electron transport chain in oxidative phosphorylation is a prime example of this principle.
Membrane Potential and Ion Gradients
The creation and maintenance of ion gradients across cellular membranes represent a crucial method for generating energy that can fuel thermodynamically unfavorable reactions. The difference in electrical potential across the membrane (membrane potential) coupled with the chemical potential due to the concentration differences of ions, like protons (H+), create a powerful driving force (proton motive force). This force is used to drive ATP synthesis through chemiosmosis.
Environmental Factors
The thermodynamic spontaneity of a reaction is also influenced by factors like temperature, pressure, and pH. While cells don't directly alter external factors like atmospheric pressure, they carefully maintain their internal environment (temperature and pH) to optimize the functioning of various metabolic pathways. This intracellular environment provides a setting that makes certain reactions more favorable than they would be under standard conditions. Deviations from standard conditions influence the actual free energy change (ΔG'), making reactions potentially more favorable than anticipated by their standard free energy change (ΔG°).
Conclusion
The ability of biological pathways to incorporate thermodynamically unfavorable reactions is a testament to the sophistication of cellular machinery. By employing strategies such as reaction coupling, manipulating reactant and product concentrations, utilizing enzyme catalysis, compartmentalization, electron carriers, and ion gradients, cells can effectively overcome the inherent energy barriers of endergonic reactions. These mechanisms ensure that the overall metabolic pathways are energetically favorable, driving the myriad of life-sustaining processes within the cell. The intricacy and elegance of this fine-tuned interplay of thermodynamics and cellular mechanisms highlight the remarkable complexity and efficiency of biological systems.
Latest Posts
Latest Posts
-
When There Is A Climate Of Mistrust Employees
Apr 02, 2025
-
Enzymes Are Important Molecules In Biochemistry That Catalyze Reactions
Apr 02, 2025
-
Return All Unused Chemicals To Their Original Containers
Apr 02, 2025
-
Which Of The Following Is An Example Of Hopelessness Theory
Apr 02, 2025
-
Running Off Track Case Study Answer Key
Apr 02, 2025
Related Post
Thank you for visiting our website which covers about How Can A Biological Pathway Contain A Thermodynamically Unfavorable Reaction . We hope the information provided has been useful to you. Feel free to contact us if you have any questions or need further assistance. See you next time and don't miss to bookmark.