Enzymes Are Important Molecules In Biochemistry That Catalyze Reactions
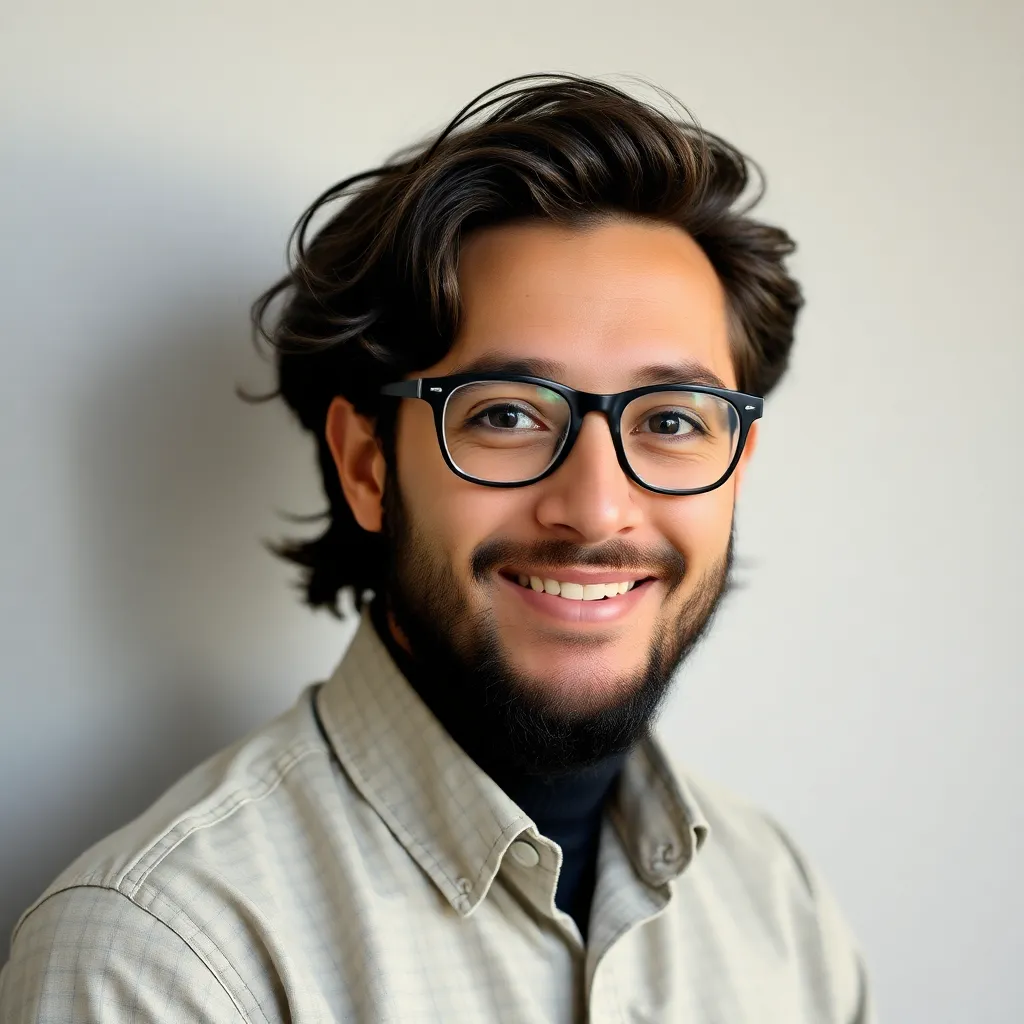
Holbox
Apr 02, 2025 · 6 min read

Table of Contents
- Enzymes Are Important Molecules In Biochemistry That Catalyze Reactions
- Table of Contents
- Enzymes: The Unsung Heroes of Biochemistry
- Understanding Enzyme Structure and Function
- The Active Site: A Precision Tool
- Enzyme Classification: A Systematic Approach
- Enzyme Kinetics: Understanding Reaction Rates
- Factors Affecting Enzyme Activity
- Enzyme Mechanisms: How Enzymes Accelerate Reactions
- The Importance of Enzymes in Metabolism
- Glycolysis: Energy Harvesting from Glucose
- Citric Acid Cycle (Krebs Cycle): Central Hub of Metabolism
- Protein Synthesis: Building the Workhorses of the Cell
- DNA Replication and Repair: Maintaining Genetic Integrity
- Enzyme Regulation: Controlling Metabolic Flux
- Enzymes in Medicine and Biotechnology
- Enzyme-based diagnostics: Measuring biomarkers
- Enzyme therapy: Treating diseases
- Industrial applications: Catalyzing reactions
- Conclusion: The Ubiquitous Role of Enzymes
- Latest Posts
- Latest Posts
- Related Post
Enzymes: The Unsung Heroes of Biochemistry
Enzymes are biological molecules, primarily proteins, that act as catalysts within living organisms. Their importance in biochemistry cannot be overstated; they are essential for virtually every metabolic process. Without enzymes, the chemical reactions necessary for life would proceed far too slowly to sustain life. This article delves into the fascinating world of enzymes, exploring their structure, function, mechanisms of action, and their crucial roles in various biochemical pathways.
Understanding Enzyme Structure and Function
Enzymes are highly specific, meaning they catalyze only one or a very limited range of chemical reactions. This specificity arises from their unique three-dimensional structures. The intricate folding of the polypeptide chains creates specific regions, known as active sites, where substrate molecules bind and the catalytic reaction occurs.
The Active Site: A Precision Tool
The active site is a crucial region within the enzyme's structure. It possesses a unique arrangement of amino acid residues that interacts with the substrate through a variety of weak forces, including hydrogen bonds, ionic interactions, van der Waals forces, and hydrophobic interactions. This precise interaction ensures that only the correct substrate can bind, and that the reaction proceeds with high efficiency.
Enzyme Classification: A Systematic Approach
Enzymes are classified into six broad categories based on the type of reaction they catalyze:
- Oxidoreductases: Catalyze oxidation-reduction reactions. These enzymes transfer electrons between molecules, often involving coenzymes like NAD+ and FAD.
- Transferases: Catalyze the transfer of functional groups (e.g., methyl, amino, phosphate groups) between molecules. Kinases, which transfer phosphate groups from ATP, are a prominent example.
- Hydrolases: Catalyze the hydrolysis of chemical bonds, breaking them down by adding water. Examples include lipases (breaking down lipids) and proteases (breaking down proteins).
- Lyases: Catalyze the addition or removal of groups to or from a molecule, often forming double bonds in the process. Decarboxylases, which remove carboxyl groups from molecules, are a common example.
- Isomerases: Catalyze the rearrangement of atoms within a molecule, converting it into an isomer.
- Ligases: Catalyze the joining of two molecules, often coupled to the hydrolysis of ATP. DNA ligase, which joins DNA fragments, is a well-known example.
Enzyme Kinetics: Understanding Reaction Rates
Enzyme kinetics studies the rates of enzyme-catalyzed reactions and the factors that influence them. The Michaelis-Menten equation is a cornerstone of enzyme kinetics, describing the relationship between substrate concentration ([S]) and reaction velocity (V). It introduces two important constants:
- K<sub>m</sub> (Michaelis constant): Represents the substrate concentration at which the reaction velocity is half of its maximum value (V<sub>max</sub>). A lower K<sub>m</sub> indicates a higher affinity of the enzyme for the substrate.
- V<sub>max</sub> (Maximum velocity): Represents the maximum rate of the reaction when the enzyme is saturated with substrate.
Factors Affecting Enzyme Activity
Several factors significantly impact enzyme activity:
- Temperature: Enzymes typically have an optimal temperature at which they function most efficiently. Higher temperatures can denature the enzyme, destroying its three-dimensional structure and activity.
- pH: Each enzyme has an optimal pH range. Changes in pH can alter the ionization state of amino acid residues in the active site, affecting substrate binding and catalysis.
- Substrate concentration: Increasing substrate concentration generally increases reaction velocity until V<sub>max</sub> is reached, at which point the enzyme is saturated.
- Enzyme concentration: Increasing enzyme concentration increases reaction velocity proportionally, assuming sufficient substrate is available.
- Inhibitors: Molecules that decrease enzyme activity. Inhibitors can be competitive (competing with the substrate for the active site) or non-competitive (binding to a site other than the active site, altering the enzyme's conformation).
Enzyme Mechanisms: How Enzymes Accelerate Reactions
Enzymes achieve remarkable rate enhancements by lowering the activation energy of the reaction. This is accomplished through various mechanisms:
- Substrate binding and orientation: The enzyme brings substrates together in the correct orientation for the reaction to occur, increasing the probability of successful collisions.
- Acid-base catalysis: Amino acid residues in the active site act as acids or bases, donating or accepting protons to facilitate the reaction.
- Covalent catalysis: The enzyme forms a temporary covalent bond with the substrate, creating a reactive intermediate that facilitates the reaction.
- Metal ion catalysis: Metal ions, often bound to the enzyme, participate directly in the catalytic mechanism. They can act as Lewis acids, accepting electron pairs, or they can stabilize charged intermediates.
The Importance of Enzymes in Metabolism
Enzymes are central to all aspects of metabolism, the sum of all chemical reactions in an organism. They orchestrate the intricate network of pathways that build, break down, and interconvert molecules. Let's examine some key examples:
Glycolysis: Energy Harvesting from Glucose
Glycolysis, the breakdown of glucose to pyruvate, is a fundamental metabolic pathway. Each step of glycolysis is catalyzed by a specific enzyme. These enzymes work in concert to efficiently extract energy from glucose, providing ATP (adenosine triphosphate), the primary energy currency of the cell.
Citric Acid Cycle (Krebs Cycle): Central Hub of Metabolism
The citric acid cycle is another crucial metabolic pathway, occurring in the mitochondria. A series of enzyme-catalyzed reactions oxidize acetyl-CoA, releasing carbon dioxide and generating high-energy electron carriers (NADH and FADH2) which fuel ATP production in oxidative phosphorylation.
Protein Synthesis: Building the Workhorses of the Cell
Protein synthesis involves a complex interplay of enzymes, including those responsible for transcription (DNA to RNA) and translation (RNA to protein). Ribosomes, themselves complex ribonucleoprotein machines, rely heavily on enzymatic activity to ensure the accurate and efficient synthesis of proteins.
DNA Replication and Repair: Maintaining Genetic Integrity
DNA replication and repair are vital processes for maintaining the integrity of the genome. A suite of enzymes, including DNA polymerases (catalyzing DNA synthesis) and nucleases (cutting DNA strands), are essential for these processes. Errors in these processes can have serious consequences, leading to mutations and diseases.
Enzyme Regulation: Controlling Metabolic Flux
The activity of enzymes is tightly regulated to ensure that metabolic pathways operate efficiently and in response to the cell's needs. Several mechanisms control enzyme activity:
- Allosteric regulation: Binding of molecules to regulatory sites (different from the active site) alters the enzyme's conformation and activity.
- Covalent modification: Reversible chemical modifications, such as phosphorylation or acetylation, can activate or inhibit enzymes.
- Enzyme synthesis and degradation: The levels of enzymes are controlled by regulating their synthesis (transcription and translation) and degradation (proteolysis).
- Compartmentalization: Enzymes are often localized within specific cellular compartments, allowing for spatial regulation of metabolic pathways.
Enzymes in Medicine and Biotechnology
Enzymes play a significant role in medicine and biotechnology. They are used in diagnostic tests, as therapeutic agents, and in industrial processes.
Enzyme-based diagnostics: Measuring biomarkers
Enzymes are used extensively in diagnostic assays to measure the levels of specific biomarkers in blood or other body fluids. For example, elevated levels of certain enzymes can indicate damage to specific organs.
Enzyme therapy: Treating diseases
Enzymes are employed therapeutically to treat a variety of diseases. For example, enzyme replacement therapy is used to treat genetic disorders involving enzyme deficiencies.
Industrial applications: Catalyzing reactions
Enzymes are widely used in industrial processes as biocatalysts. They are employed in various applications, such as food processing, textile manufacturing, and the production of pharmaceuticals.
Conclusion: The Ubiquitous Role of Enzymes
Enzymes are fundamental to life, catalyzing the myriad of reactions that sustain living organisms. Their remarkable specificity, efficiency, and regulation make them indispensable components of all metabolic pathways. Understanding enzyme structure, function, and regulation is vital for comprehending the complexities of biological systems and developing innovative applications in medicine, biotechnology, and other fields. Further research into enzyme mechanisms and engineering continues to unlock their potential for solving various challenges facing humanity. The world of enzymes is vast and continuously revealing new insights, highlighting their ongoing importance in scientific exploration and technological advancement.
Latest Posts
Latest Posts
-
All Queries Belong To A Locale
Apr 06, 2025
-
To Analyze The Characteristics And Performance Of The Brakes
Apr 06, 2025
-
A Is An Electronic Document That Can Contain Text
Apr 06, 2025
-
Unlike Other Democracies The United States
Apr 06, 2025
-
For Managers Good Listening Begins With
Apr 06, 2025
Related Post
Thank you for visiting our website which covers about Enzymes Are Important Molecules In Biochemistry That Catalyze Reactions . We hope the information provided has been useful to you. Feel free to contact us if you have any questions or need further assistance. See you next time and don't miss to bookmark.