Genes Turn On Or Off To Regulate The Activity Of
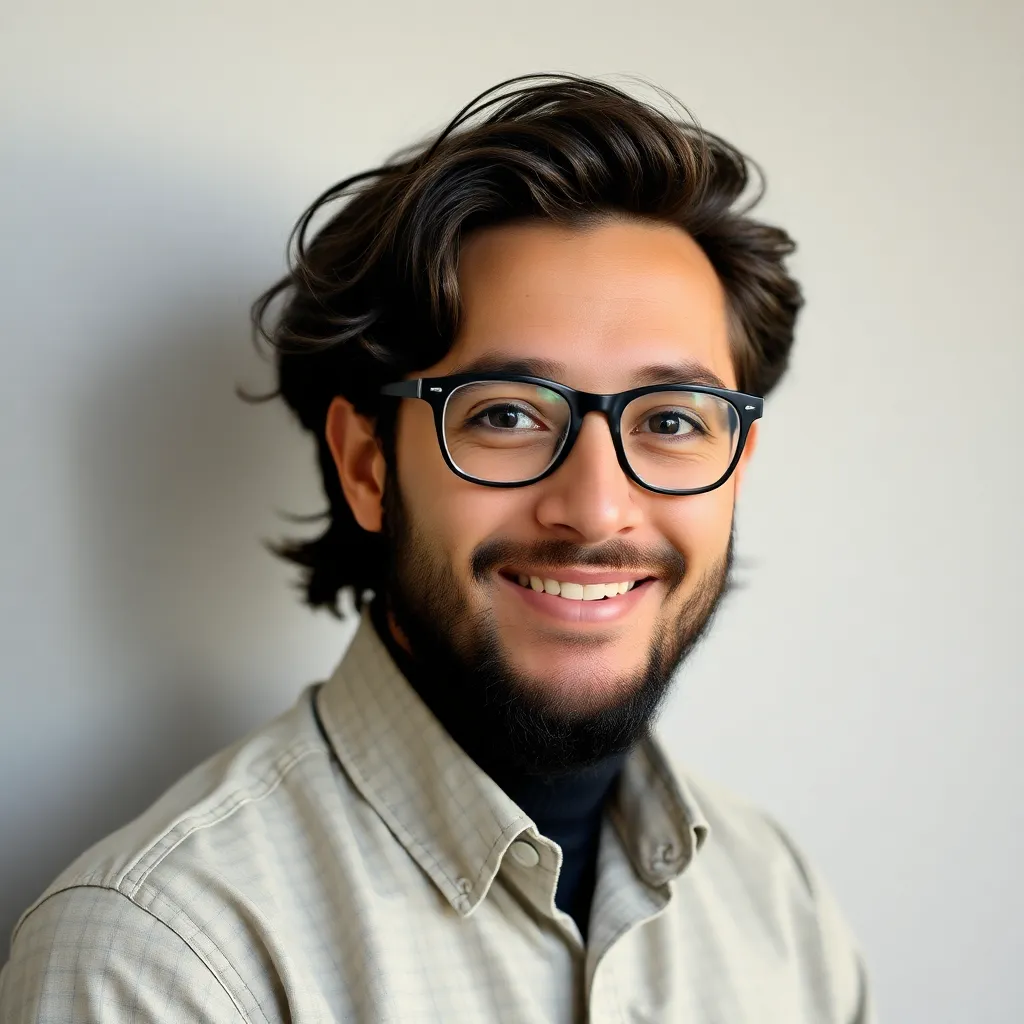
Holbox
Mar 29, 2025 · 6 min read
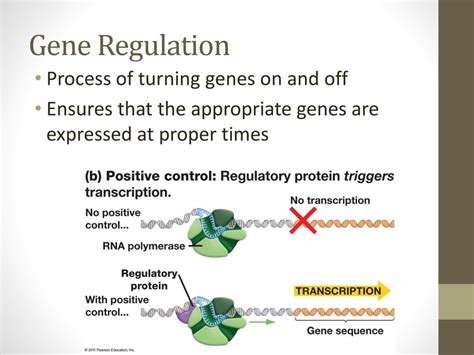
Table of Contents
- Genes Turn On Or Off To Regulate The Activity Of
- Table of Contents
- Genes Turn On or Off: The Intricate Dance of Gene Regulation
- The Central Dogma: From DNA to Protein
- Transcription: The First Gatekeeper
- Epigenetics: Modifying the Chromatin Landscape
- RNA Processing and Splicing: Fine-Tuning Gene Expression
- RNA Interference (RNAi): Silencing Genes with Small RNAs
- Protein Degradation: The Final Control Point
- Clinical Significance of Gene Regulation
- Conclusion: A Complex and Dynamic Process
- Latest Posts
- Latest Posts
- Related Post
Genes Turn On or Off: The Intricate Dance of Gene Regulation
The human body, a marvel of complexity, is orchestrated by trillions of cells, each performing specific functions with remarkable precision. This intricate cellular choreography isn't haphazard; it's meticulously directed by the precise regulation of gene activity. Genes, the fundamental units of heredity, don't simply exist in a constant state of "on." Instead, they engage in a dynamic dance of activation and repression, turning on and off in response to internal and external signals. This precise control mechanism is crucial for development, growth, homeostasis, and response to environmental changes. Understanding how genes are regulated is key to comprehending life itself, and opens doors to advancements in medicine and biotechnology.
The Central Dogma: From DNA to Protein
Before delving into the mechanisms of gene regulation, it's vital to revisit the central dogma of molecular biology. This dogma outlines the flow of genetic information: DNA (deoxyribonucleic acid) is transcribed into RNA (ribonucleic acid), which is then translated into protein. Proteins are the workhorses of the cell, responsible for a vast array of functions, including catalyzing metabolic reactions (enzymes), providing structural support, and mediating cellular signaling. The regulation of gene expression occurs at multiple steps within this pathway, allowing for fine-tuned control over protein production.
Transcription: The First Gatekeeper
Transcription, the process of creating an RNA copy of a DNA sequence, is the primary point of gene regulation. The key player here is RNA polymerase, an enzyme that binds to DNA and synthesizes RNA. However, RNA polymerase doesn't simply bind to any DNA sequence; it requires specific DNA sequences called promoters to initiate transcription. Promoters are located upstream of the gene they regulate and act as binding sites for RNA polymerase and other regulatory proteins called transcription factors.
Transcription Factors: The Master Regulators:
Transcription factors are proteins that can either enhance or repress the binding of RNA polymerase to the promoter. Activator proteins bind to specific DNA sequences called enhancer regions and help recruit RNA polymerase to the promoter, stimulating transcription. Conversely, repressor proteins bind to silencer regions, blocking RNA polymerase access to the promoter and inhibiting transcription.
The interaction between transcription factors and their DNA binding sites is highly specific. Slight changes in the DNA sequence can dramatically alter the binding affinity of a transcription factor, effectively turning a gene on or off. This specificity is further enhanced by the cooperative binding of multiple transcription factors, creating complex regulatory networks.
Epigenetics: Modifying the Chromatin Landscape
The DNA within a cell isn't simply a naked strand; it's intricately packaged with proteins into a structure called chromatin. The structure of chromatin significantly influences gene accessibility to RNA polymerase. Highly condensed chromatin, called heterochromatin, makes genes inaccessible for transcription, effectively silencing them. Loosely packed chromatin, called euchromatin, allows for easy access and active transcription.
Epigenetic modifications are heritable alterations in gene expression that do not involve changes to the underlying DNA sequence. These modifications include:
- DNA methylation: The addition of a methyl group (CH3) to a cytosine base, typically leading to gene silencing.
- Histone modification: Chemical modifications (acetylation, methylation, phosphorylation) to histone proteins, which alter chromatin structure and gene expression.
These epigenetic modifications can be influenced by environmental factors, such as diet and stress, and can have long-lasting effects on gene expression. They play a crucial role in development, cellular differentiation, and disease susceptibility.
RNA Processing and Splicing: Fine-Tuning Gene Expression
Even after transcription, gene expression can be further regulated. In eukaryotic cells, the newly synthesized RNA molecule undergoes several processing steps before it can be translated into protein. This includes:
- Capping: Adding a modified guanine nucleotide to the 5' end of the RNA molecule, protecting it from degradation and aiding in translation initiation.
- Splicing: Removing non-coding regions called introns and joining the coding regions called exons. Alternative splicing can generate multiple protein isoforms from a single gene, expanding the proteome's diversity.
- Polyadenylation: Adding a poly(A) tail to the 3' end, stabilizing the RNA molecule and enhancing its translation.
These RNA processing steps can be regulated, leading to variations in the amount and type of protein produced from a single gene.
RNA Interference (RNAi): Silencing Genes with Small RNAs
RNA interference (RNAi) is a powerful mechanism for silencing gene expression at the post-transcriptional level. Small RNA molecules, such as microRNAs (miRNAs) and short interfering RNAs (siRNAs), bind to complementary sequences in mRNA molecules. This binding can lead to:
- mRNA degradation: The targeted mRNA molecule is broken down, preventing translation.
- Translational repression: The ribosome is prevented from translating the mRNA molecule.
RNAi plays important roles in development, immunity, and defense against viruses. It's also a valuable tool for researchers studying gene function and developing new therapies.
Protein Degradation: The Final Control Point
Even after a protein is synthesized, its activity can be regulated by controlling its degradation. The ubiquitin-proteasome system is a major pathway for protein degradation. Ubiquitin, a small protein, is attached to target proteins, marking them for degradation by the proteasome, a protein complex that breaks down unwanted proteins. The regulation of ubiquitination is a crucial mechanism for controlling protein levels and activity.
Clinical Significance of Gene Regulation
Dysregulation of gene expression is implicated in a wide range of human diseases, including cancer, neurological disorders, and metabolic diseases. Cancer, for instance, is often characterized by uncontrolled cell growth and proliferation, frequently driven by mutations or epigenetic alterations that disrupt gene regulation. Understanding the mechanisms underlying these dysregulations is crucial for developing effective diagnostic tools and therapeutic strategies.
Targeted Therapies: The advancements in understanding gene regulation have led to the development of targeted therapies that specifically inhibit or enhance the activity of key regulatory molecules. For example, drugs that inhibit specific kinases involved in cell signaling pathways have shown remarkable success in treating certain cancers.
Gene Therapy: Gene therapy aims to correct genetic defects by introducing functional genes into cells. While still under development, gene therapy holds immense promise for treating genetic disorders. Understanding gene regulation is critical for designing effective gene therapy strategies.
Conclusion: A Complex and Dynamic Process
Gene regulation is a complex and dynamic process involving a cascade of events, from transcription initiation to protein degradation. The intricate interplay of transcription factors, epigenetic modifications, RNA processing, RNAi, and protein degradation ensures precise control over gene expression, allowing cells to respond appropriately to internal and external stimuli. Disruptions in this finely tuned system can lead to various diseases, highlighting the importance of ongoing research in this crucial area of biology. The future of medicine and biotechnology hinges on our ability to harness the power of gene regulation for therapeutic purposes, opening exciting new avenues for disease prevention and treatment. Further research into the intricate details of gene regulation will undoubtedly reveal even more complex and fascinating mechanisms governing life itself.
Latest Posts
Latest Posts
-
Chemical Reactions Occur As A Result Of
Apr 01, 2025
-
Stage Theories Hold That The Sequence Of Development Is
Apr 01, 2025
-
Which Occupation Would Most Likely Be Involved In Genome Mapping
Apr 01, 2025
-
A Critical Analysis Based On Heuristics Will Lead To
Apr 01, 2025
-
Predict The Major Organic Product Of The Following Reaction
Apr 01, 2025
Related Post
Thank you for visiting our website which covers about Genes Turn On Or Off To Regulate The Activity Of . We hope the information provided has been useful to you. Feel free to contact us if you have any questions or need further assistance. See you next time and don't miss to bookmark.