Facilitated Diffusion Requires A Specific Transporter For A Specific Molecule
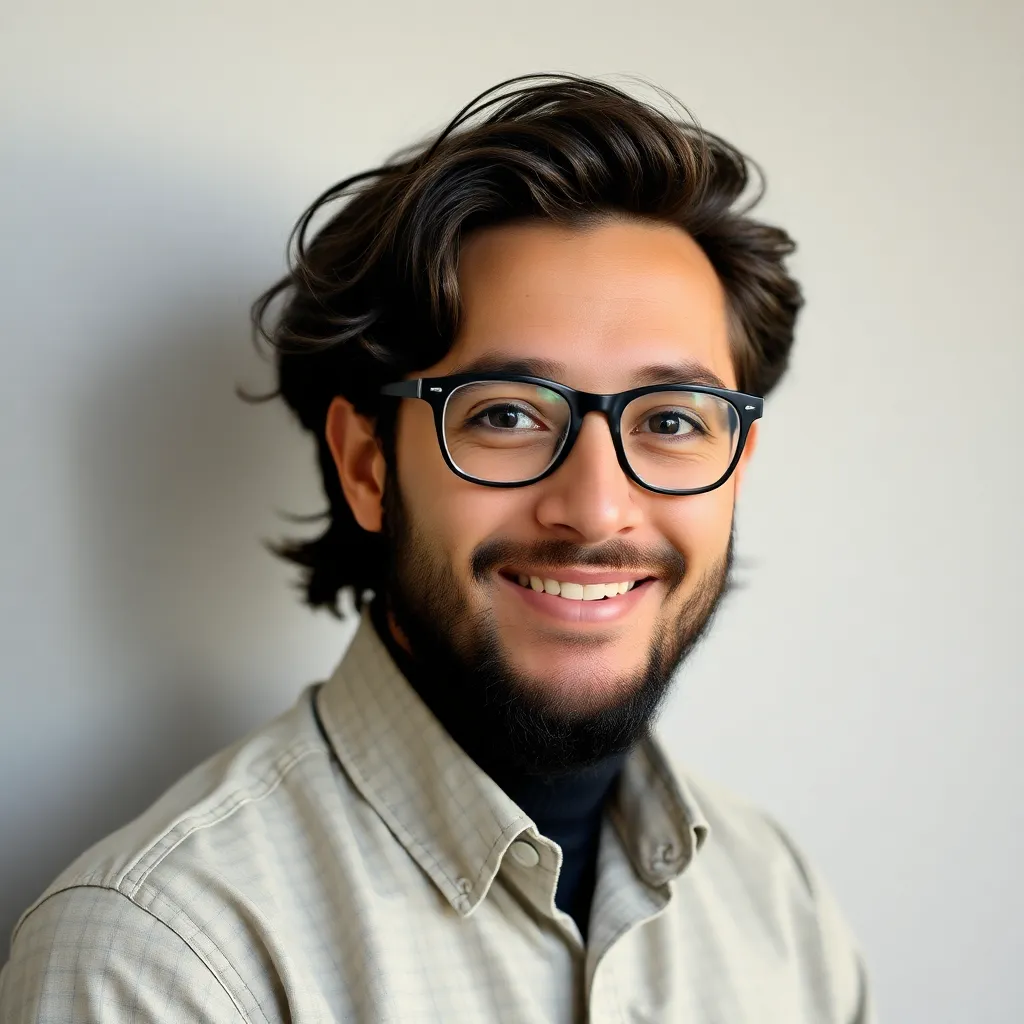
Holbox
Apr 17, 2025 · 7 min read
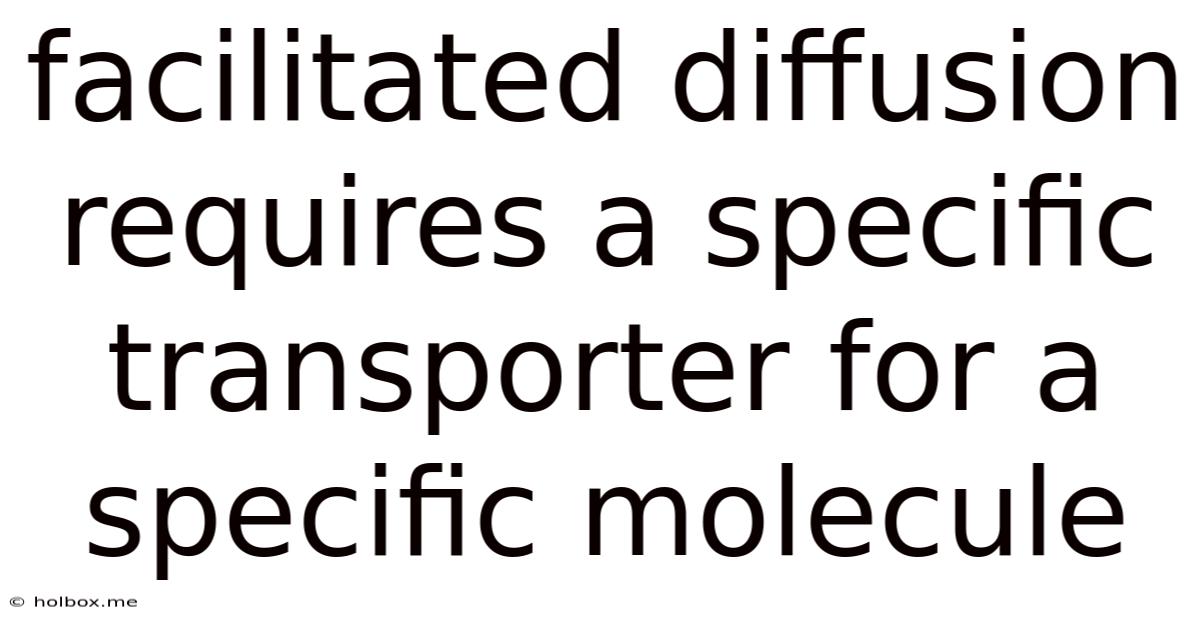
Table of Contents
- Facilitated Diffusion Requires A Specific Transporter For A Specific Molecule
- Table of Contents
- Facilitated Diffusion: The Specific Transporter, the Specific Molecule
- The Nature of Membrane Transporters
- Channel Proteins: Selective Gates
- Carrier Proteins: Conformational Changes
- The Specificity Principle in Action: Examples
- Glucose Transport: GLUT Transporters
- Amino Acid Transport: Diverse Transporter Families
- Ion Channels: Maintaining Cellular Electrophysiology
- The Importance of Transporter Specificity
- Preventing Toxic Accumulation
- Maintaining Concentration Gradients
- Regulating Metabolic Pathways
- Responding to Environmental Changes
- Consequences of Transporter Dysfunction
- Genetic Defects: Inherited Diseases
- Acquired Defects: Drug Interactions & Disease States
- Therapeutic Targets: Drug Development
- Conclusion: The Intricate Dance of Specificity
- Latest Posts
- Latest Posts
- Related Post
Facilitated Diffusion: The Specific Transporter, the Specific Molecule
Facilitated diffusion is a passive transport process that moves molecules across cell membranes with the assistance of membrane proteins. Unlike simple diffusion, which relies solely on the concentration gradient, facilitated diffusion utilizes specific transporter proteins to greatly enhance the rate of movement of molecules across the otherwise impermeable lipid bilayer. This process is crucial for the efficient uptake and release of essential molecules, including sugars, amino acids, ions, and nucleotides, within living organisms. Crucially, facilitated diffusion requires a specific transporter for a specific molecule, a feature that underscores the remarkable specificity and regulation inherent in cellular transport mechanisms.
The Nature of Membrane Transporters
The heart of facilitated diffusion lies in the diverse array of membrane transport proteins. These proteins are embedded within the phospholipid bilayer and act as selective channels or carriers, allowing specific molecules to traverse the membrane. They achieve this remarkable selectivity through a complex interplay of protein structure and molecular interactions.
Channel Proteins: Selective Gates
Channel proteins form hydrophilic pores that span the membrane, providing a pathway for the passage of specific ions or small polar molecules. These channels are often gated, meaning their opening and closing is regulated by specific stimuli, including changes in membrane potential, ligand binding, or mechanical forces. This regulation allows cells to precisely control the influx and efflux of ions, maintaining crucial ionic gradients that underpin many cellular processes. For instance, voltage-gated ion channels in neurons are critical for the propagation of nerve impulses.
Specificity in channel proteins arises from the precise arrangement of amino acid residues lining the pore. These residues interact with the transported molecule through electrostatic interactions, hydrogen bonds, or van der Waals forces, ensuring that only molecules with a complementary shape and charge can pass through. Any slight deviation in structure can prevent passage, emphasizing the high degree of selectivity.
Carrier Proteins: Conformational Changes
Carrier proteins, also known as permeases or transporters, undergo conformational changes to move molecules across the membrane. Unlike channel proteins that simply form a pore, carrier proteins bind their specific substrate on one side of the membrane, undergo a conformational shift, and then release the substrate on the other side. This process is highly specific, as the binding site on the carrier protein is meticulously tailored to the structure of the transported molecule.
The binding affinity of the carrier protein for the specific molecule is crucial for the efficiency of transport. High affinity ensures efficient binding even at low concentrations, while the conformational change facilitates movement against the otherwise unfavorable energetic landscape of the membrane. The release of the molecule on the other side of the membrane is typically driven by the concentration gradient.
The Specificity Principle in Action: Examples
The principle of "a specific transporter for a specific molecule" is not just a theoretical concept; it is a fundamental aspect of cellular function, manifested in a multitude of examples.
Glucose Transport: GLUT Transporters
Glucose, a primary energy source for cells, is transported across cell membranes by a family of glucose transporter proteins (GLUTs). Different GLUT isoforms exhibit varying substrate affinities and tissue-specific expression patterns. GLUT1, for example, is expressed in most tissues and facilitates basal glucose uptake, while GLUT4, found predominantly in muscle and adipose tissue, is insulin-responsive, mediating glucose uptake in response to hormonal signals. Each GLUT transporter is highly specific for D-glucose, demonstrating the principle of specific transporter-substrate interaction. Other sugars, even closely related isomers, are not efficiently transported by GLUTs.
Amino Acid Transport: Diverse Transporter Families
Amino acids, the building blocks of proteins, are transported across cell membranes by a diverse array of transporter families, each exhibiting remarkable specificity for particular amino acid types or groups. Some transporters are specific for neutral amino acids, while others selectively transport acidic or basic amino acids. This selectivity is critical for maintaining balanced amino acid levels within cells and preventing the accumulation of toxic metabolites. The exquisite specificity of these transporters ensures the efficient uptake of essential amino acids while preventing the entry of potentially harmful compounds.
Ion Channels: Maintaining Cellular Electrophysiology
Ion channels exemplify the specificity of transporter-molecule interactions in the context of maintaining cellular electrophysiology. Voltage-gated sodium channels, for example, are highly selective for sodium ions (Na+), exhibiting a much lower permeability to potassium ions (K+) or other cations. This selectivity is crucial for the rapid depolarization phase of action potentials in neurons and muscle cells. Similarly, potassium channels are highly selective for K+, facilitating repolarization and maintaining resting membrane potential. The precise tuning of ion channel selectivity is essential for proper electrical signaling in excitable cells.
The Importance of Transporter Specificity
The specificity of transporters is not merely a quirk of biological design; it is a fundamental requirement for cellular homeostasis and function.
Preventing Toxic Accumulation
Selective transport prevents the accumulation of potentially harmful molecules within cells. If transporters lacked specificity, cells could inadvertently accumulate toxic substances, leading to cellular dysfunction and even cell death. The specificity of transporters acts as a crucial safeguard, ensuring that only essential molecules enter the cell.
Maintaining Concentration Gradients
The specificity of transporters is critical for maintaining concentration gradients across cell membranes. These gradients are essential for many cellular processes, including energy production, signal transduction, and nutrient uptake. If transporters were non-specific, these gradients would dissipate, disrupting crucial cellular functions.
Regulating Metabolic Pathways
The regulated transport of specific molecules through transporters allows cells to precisely control metabolic pathways. The selective uptake of substrates and the release of products ensure that metabolic reactions proceed efficiently and effectively, avoiding the wasteful accumulation of intermediates or the depletion of essential precursors.
Responding to Environmental Changes
The expression and activity of specific transporters can be modulated in response to environmental changes. For instance, cells exposed to nutrient deprivation may upregulate the expression of transporters for specific nutrients to ensure survival. This adaptability demonstrates the importance of transporter specificity in allowing cells to respond and adapt to changing conditions.
Consequences of Transporter Dysfunction
Given the critical roles of membrane transporters in cellular physiology, malfunctions in transporter function can lead to a range of pathological conditions.
Genetic Defects: Inherited Diseases
Mutations in genes encoding membrane transporters can cause inherited diseases. For example, defects in glucose transporters (GLUTs) can lead to glucose intolerance and hypoglycemia. Similarly, mutations in amino acid transporters can result in disorders of amino acid metabolism, leading to neurological and developmental abnormalities. These inherited diseases highlight the crucial role of specific transporters in maintaining normal cellular function.
Acquired Defects: Drug Interactions & Disease States
Transporter dysfunction can also result from acquired factors, including drug interactions and disease states. Many drugs interact with membrane transporters, either inhibiting their activity or increasing their function. This can have profound effects on drug absorption, distribution, metabolism, and excretion (ADME), leading to drug interactions and adverse effects. Furthermore, numerous diseases, including cancer and heart disease, are associated with altered transporter expression or function.
Therapeutic Targets: Drug Development
The crucial role of membrane transporters in health and disease makes them attractive targets for drug development. Many drugs are designed to modulate the activity of specific transporters to treat various diseases, including cancer, diabetes, and infectious diseases. Understanding the specificity of transporter-molecule interactions is critical for designing effective and targeted therapies.
Conclusion: The Intricate Dance of Specificity
Facilitated diffusion, mediated by specific transporters for specific molecules, represents a cornerstone of cellular life. The exquisite specificity of these transporters underscores the complexity and precision of cellular mechanisms. From the regulated uptake of glucose to the precise control of ion concentrations, the interaction between transporters and their substrates exemplifies nature's elegance in achieving efficient and controlled transport across biological membranes. Understanding this intricate dance of specificity is not only crucial for appreciating the fundamentals of cellular biology but also for developing effective therapies for a broad range of diseases. The ongoing research into these fascinating protein machines continues to reveal new insights into their mechanisms and the crucial roles they play in health and disease. Future studies promise further advancements in our understanding of facilitated diffusion and its implications for medicine and biotechnology.
Latest Posts
Latest Posts
-
Which Of These Statements Concerning Traditional Iras Is Correct
May 07, 2025
-
A Patient Has A Rapid Irregular Wide Complex Tachycardia
May 07, 2025
-
The Axes On A Perceptual Map Are
May 07, 2025
-
Ap Literature Unit 7 Progress Check Mcq Answers
May 07, 2025
-
Which Statement Is Supported By The Data Table
May 07, 2025
Related Post
Thank you for visiting our website which covers about Facilitated Diffusion Requires A Specific Transporter For A Specific Molecule . We hope the information provided has been useful to you. Feel free to contact us if you have any questions or need further assistance. See you next time and don't miss to bookmark.