Design A Synthesis Of 4-hexanolide From 4-oxohexanal
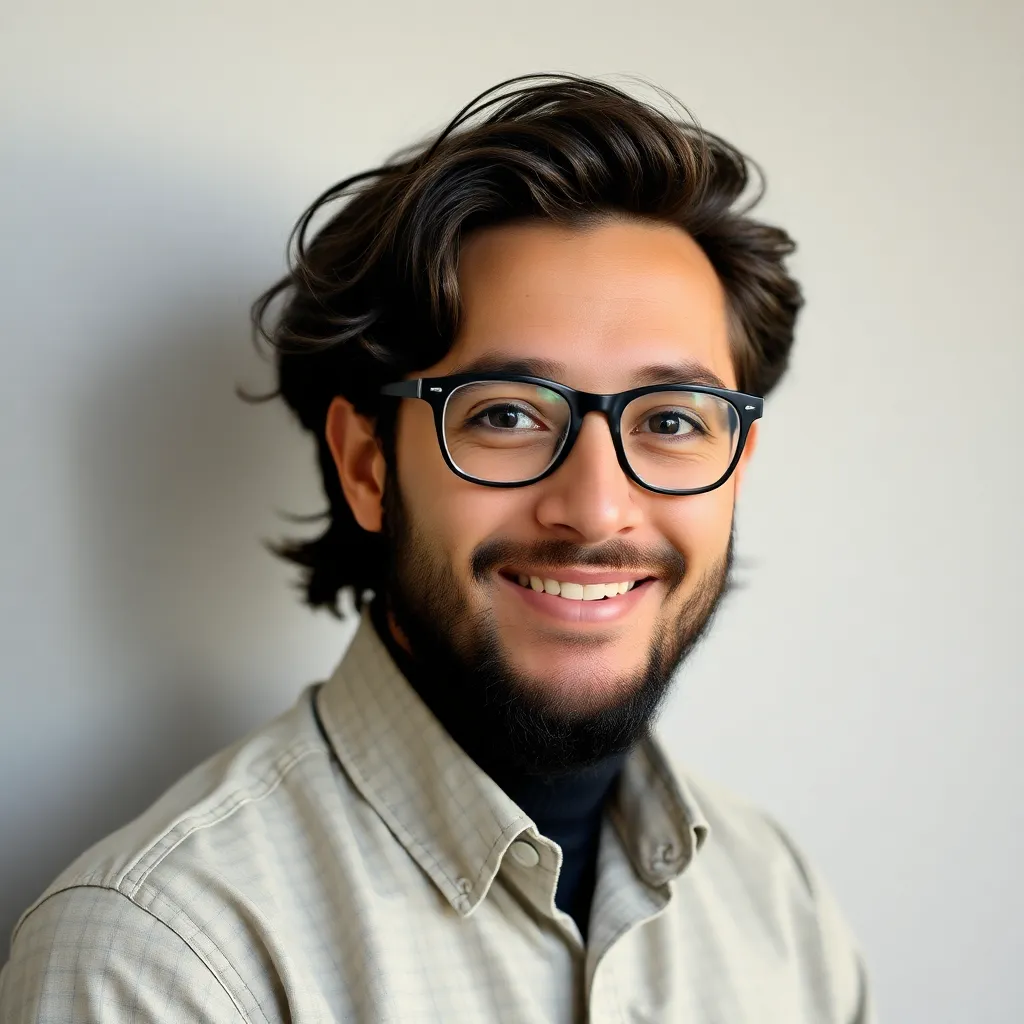
Holbox
Apr 04, 2025 · 6 min read

Table of Contents
- Design A Synthesis Of 4-hexanolide From 4-oxohexanal
- Table of Contents
- Designing a Synthesis of 4-Hexanolide from 4-Oxohexanal: A Comprehensive Guide
- Understanding the Challenge: Aldehyde to Lactone Conversion
- Proposed Synthetic Route: A Step-by-Step Approach
- Alternative Synthetic Routes: Exploring Other Possibilities
- Conclusion: A Synthetic Puzzle Solved
- Latest Posts
- Latest Posts
- Related Post
Designing a Synthesis of 4-Hexanolide from 4-Oxohexanal: A Comprehensive Guide
The synthesis of 4-hexanolide from 4-oxohexanal presents a fascinating challenge in organic chemistry, demanding a strategic approach to navigate the transformation of an aldehyde into a cyclic ester (lactone). This article will delve into a detailed, multi-faceted analysis of potential synthetic routes, exploring various reaction mechanisms, reagents, and considerations for optimizing yield and selectivity. We'll examine the challenges inherent in this transformation and propose a robust, step-by-step synthesis, emphasizing practical considerations for a successful outcome.
Understanding the Challenge: Aldehyde to Lactone Conversion
The core challenge lies in converting the linear 4-oxohexanal molecule into the cyclic structure of 4-hexanolide. This requires a careful orchestration of several key steps:
- Formation of a hydroxyl group: The aldehyde group must be functionalized to create a hydroxyl group (-OH) at the appropriate position.
- Intramolecular esterification: This hydroxyl group then needs to react with the carboxylic acid to form the ester linkage, creating the cyclic lactone structure.
Several reaction pathways can achieve this transformation, each with its own advantages and disadvantages regarding yield, selectivity, and practicality. Let's explore some of these options.
Proposed Synthetic Route: A Step-by-Step Approach
This route focuses on a strategy utilizing a Baeyer-Villiger oxidation followed by a selective reduction to generate the necessary hydroxyl group for lactonization. The synthetic route is presented as follows:
Step 1: Baeyer-Villiger Oxidation
This crucial step involves the oxidation of the keto-aldehyde using a suitable peroxyacid, such as m-chloroperoxybenzoic acid (mCPBA). The peroxyacid acts as an electrophile, attacking the carbonyl carbon of the ketone group. This generates an intermediate that subsequently undergoes rearrangement, resulting in the formation of a lactone and a carboxylic acid. However, in this case, we are aiming for a specific regioselectivity. The ketone needs to be oxidized to the lactone, not the aldehyde. This requires careful consideration of reaction conditions and choice of peroxyacid.
Reaction Mechanism:
The Baeyer-Villiger oxidation mechanism involves the following steps:
- Nucleophilic attack of the peroxyacid oxygen on the carbonyl carbon.
- Formation of a tetrahedral intermediate.
- Migration of the alkyl group adjacent to the carbonyl group (in this case, a regio-selective migration towards the aldehyde).
- Collapse of the intermediate, resulting in the formation of the lactone and a carboxylic acid byproduct.
Optimization Considerations:
- Solvent Selection: The choice of solvent plays a crucial role in influencing the reaction rate and selectivity. Dichloromethane (DCM) is often preferred due to its inertness and its ability to dissolve both the substrate and the peroxyacid.
- Temperature Control: Maintaining a low temperature, ideally around 0°C, can minimize the formation of undesired byproducts.
- Stoichiometry: Careful control of the stoichiometry of mCPBA is essential to achieve high conversion without excess reagent causing side reactions. A slight excess is often necessary, but significant excess should be avoided.
Step 2: Selective Reduction of the Aldehyde
After the Baeyer-Villiger oxidation, we're left with a lactone containing a carboxylic acid functionality, which should undergo reduction to alcohol for subsequent lactonization. The aldehyde, on the other hand, is not suitable for direct esterification to the desired lactone structure. Hence, we must have a selective reduction, leaving the newly formed lactone untouched. This presents a challenge because aldehydes are generally more reactive towards reduction than carboxylic acids.
Several reducing agents can be used for selective aldehyde reduction:
- Sodium Borohydride (NaBH₄): This is a mild reducing agent capable of reducing aldehydes to primary alcohols without affecting esters.
- Lithium Aluminum Hydride (LiAlH₄): This is a much stronger reducing agent and should be carefully avoided because of its high reactivity. In the presence of LiAlH4, both the aldehyde and the ester moiety will be reduced.
Reaction Mechanism (NaBH₄):
Sodium borohydride acts as a nucleophile, attacking the electrophilic carbonyl carbon of the aldehyde. This results in the formation of a tetrahedral intermediate, which then collapses to yield the primary alcohol.
Optimization Considerations:
- Solvent Selection: Methanol or ethanol are commonly used solvents for NaBH₄ reductions.
- Temperature Control: The reaction is usually carried out at room temperature or below to control the rate of reduction and minimize side reactions.
- Workup Procedure: A careful workup procedure is essential to ensure the complete removal of excess reducing agent and other impurities.
Step 3: Intramolecular Esterification (Lactonization)
With both the hydroxyl and the carboxyl groups in place, the final step is to catalyze the formation of the cyclic ester. This step can be achieved using a variety of methods, including acid catalysis or using coupling reagents. However, given the presence of the sensitive lactone structure, acid catalysis is likely to be too harsh.
A more suitable approach would utilize a coupling reagent such as DCC (dicyclohexylcarbodiimide). DCC activates the carboxylic acid, facilitating nucleophilic attack by the hydroxyl group and subsequent lactonization.
Reaction Mechanism (DCC-mediated lactonization):
DCC reacts with the carboxylic acid, forming an activated ester intermediate. This activated ester then undergoes intramolecular nucleophilic attack by the hydroxyl group, leading to the formation of the cyclic ester (4-hexanolide). The dicyclohexylurea byproduct is typically removed through filtration.
Optimization Considerations:
- Solvent Selection: Aprotic solvents like dichloromethane or dimethylformamide (DMF) are typically used.
- Temperature Control: The reaction is usually carried out at room temperature or slightly elevated temperatures to optimize the reaction rate.
- Catalyst: A catalytic amount of DMAP (4-dimethylaminopyridine) is often added to increase the reaction rate.
Alternative Synthetic Routes: Exploring Other Possibilities
While the proposed route offers a plausible and relatively efficient pathway, other synthetic strategies could be explored. These routes may involve different combinations of reactions and reagents, potentially offering alternative advantages or circumventing some of the challenges encountered in the primary route. For instance, a Wittig reaction could be utilized for the initial steps to construct the carbon skeleton more directly. However, the challenges associated with the selectivity of the reaction and functional group compatibility remain critical.
Conclusion: A Synthetic Puzzle Solved
The synthesis of 4-hexanolide from 4-oxohexanal requires a meticulous approach, taking into account the regioselectivity of each reaction step and functional group compatibility. The proposed route, employing a Baeyer-Villiger oxidation followed by a selective reduction and lactonization, provides a viable pathway. However, careful optimization of reaction conditions, choice of reagents, and workup procedures are crucial for achieving high yields and selectivity. Further exploration of alternative synthetic strategies remains a valuable area of investigation, potentially leading to improved efficiency and practicality in the synthesis of this important lactone. The entire process highlights the importance of strategic thinking and practical skill in organic synthesis, demonstrating how a seemingly simple transformation requires a deep understanding of reaction mechanisms and careful experimental design.
Latest Posts
Latest Posts
-
How Can You Refine Your Content Distribution Strategy
Apr 14, 2025
-
Density Of Water In Lb In3
Apr 14, 2025
-
How Are Electric Field And Temperature Field Different
Apr 14, 2025
-
Decide State Equivalence With Implication Table
Apr 14, 2025
-
Which Term Best Describes The Angle Below
Apr 14, 2025
Related Post
Thank you for visiting our website which covers about Design A Synthesis Of 4-hexanolide From 4-oxohexanal . We hope the information provided has been useful to you. Feel free to contact us if you have any questions or need further assistance. See you next time and don't miss to bookmark.