How Are Electric Field And Temperature Field Different
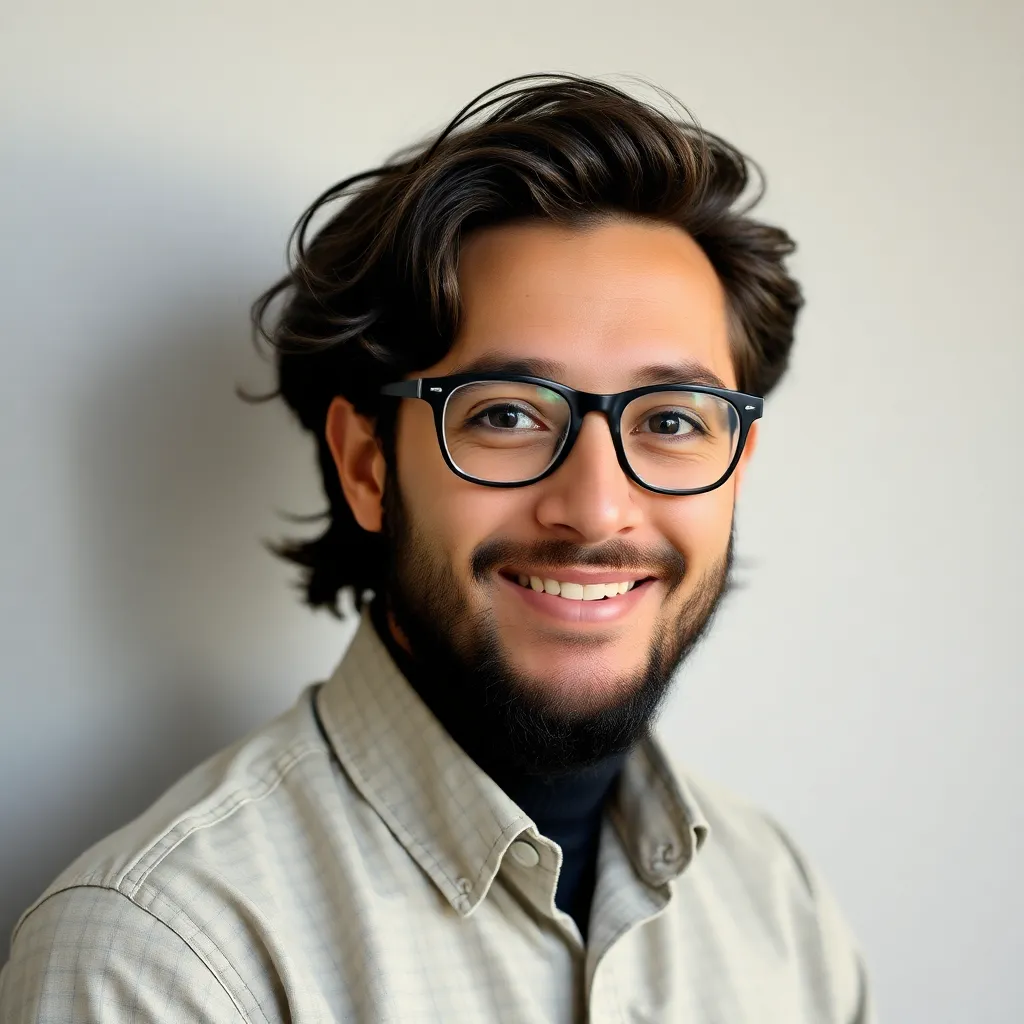
Holbox
Apr 14, 2025 · 6 min read

Table of Contents
- How Are Electric Field And Temperature Field Different
- Table of Contents
- How Are Electric Fields and Temperature Fields Different?
- Fundamental Nature: Force vs. Energy
- Visualizing the Difference
- Governing Equations and Principles: Maxwell vs. Fourier
- Material Dependence: Conductivity Matters
- Interactions and Effects: Forces vs. Heat Transfer
- Examples of Differences in Action
- Measurement and Instrumentation: Different Tools
- Applications: A Wide Spectrum
- Overlapping Applications: Thermoelectric Effects
- Conclusion: Distinct but Interrelated Fields
- Latest Posts
- Latest Posts
- Related Post
How Are Electric Fields and Temperature Fields Different?
Understanding the differences between electric fields and temperature fields is crucial in various scientific and engineering disciplines. While both are physical fields that describe properties distributed in space, their fundamental nature, interactions, and effects differ significantly. This article will delve into a comprehensive comparison, highlighting their key distinctions and exploring the underlying principles governing their behavior.
Fundamental Nature: Force vs. Energy
The most fundamental difference lies in their inherent nature. An electric field is a vector field that describes the force experienced by a charged particle at any point in space. This force, governed by Coulomb's law, is proportional to the charge of the particle and the strength of the electric field at that location. The direction of the force is along the field lines, pointing from positive to negative charges. In simpler terms, an electric field represents the influence a charge exerts on its surroundings, creating a region where other charges experience a force.
A temperature field, on the other hand, is a scalar field describing the temperature at each point in space. Temperature is a measure of the average kinetic energy of the particles within a system. Unlike an electric field, a temperature field doesn't directly exert a force. Instead, it dictates the direction of heat flow, from regions of higher temperature to regions of lower temperature, a principle governed by the second law of thermodynamics. Temperature represents the internal energy state of a system, not a direct force-inducing phenomenon.
Visualizing the Difference
Imagine placing a small, positively charged particle within an electric field. It will immediately experience a force, accelerating in the direction of the field lines. Now, imagine placing a thermometer within a temperature field. The thermometer will simply register the temperature at its location; it won't experience any force due to the temperature gradient itself. The heat transfer will be a consequence of the temperature difference, leading to a flow of energy (heat) rather than a direct force.
Governing Equations and Principles: Maxwell vs. Fourier
The behavior of electric fields is described by Maxwell's equations, a set of four partial differential equations that relate electric and magnetic fields to their sources (charges and currents). These equations elegantly capture the dynamics of electromagnetic phenomena, encompassing everything from static electric fields to electromagnetic waves. The presence of charges and currents fundamentally shapes the electric field, leading to its complex behavior.
Temperature fields, however, are primarily governed by the heat equation, a partial differential equation derived from Fourier's law of heat conduction. This equation relates the rate of change of temperature to the spatial distribution of the temperature gradient and the thermal conductivity of the material. Unlike Maxwell's equations, the heat equation doesn't directly involve charges or currents; it focuses on energy transfer in the form of heat. The behavior of a temperature field depends largely on the material properties (like thermal conductivity and specific heat capacity) and the boundary conditions.
Material Dependence: Conductivity Matters
A key difference lies in the material dependence of both fields. Electric fields can exist in a vacuum, as demonstrated by the electric field surrounding a point charge in free space. The material's permittivity influences the electric field's strength, but the field itself can exist independently of the material.
Temperature fields, however, are intrinsically linked to the material's properties. A temperature field in a vacuum is meaningless; temperature is a property of matter. The thermal conductivity of a material plays a critical role in determining the rate of heat flow and the shape of the temperature field. Different materials respond differently to heat transfer, shaping the temperature field accordingly.
Interactions and Effects: Forces vs. Heat Transfer
Electric fields exert forces on charged particles, leading to various phenomena such as electric currents, electrostatic attraction or repulsion, and electromagnetic radiation. The interaction of electric fields with matter leads to diverse effects, from simple charging to complex electrical phenomena in circuits and devices.
Temperature fields, on the other hand, don't directly exert forces; instead, they drive heat transfer through conduction, convection, and radiation. Heat transfer leads to changes in temperature distribution, phase transitions (e.g., melting, boiling), and thermal expansion or contraction. The interactions of temperature fields with matter lead to changes in the material's physical and chemical properties.
Examples of Differences in Action
Consider a charged capacitor. The electric field between its plates is responsible for storing energy and exerting forces on any charged particles placed within the field. Now, consider a heated metal rod. The temperature field within the rod leads to heat transfer from the hotter end to the colder end, eventually leading to a more uniform temperature distribution. The electric field exerts a force, whereas the temperature field drives energy transfer.
Measurement and Instrumentation: Different Tools
Measuring electric fields and temperature fields requires distinct instruments and techniques. Electric fields are typically measured using devices like electrostatic voltmeters or electric field mills, which detect the force exerted on a charged probe or the displacement of charged particles within the field.
Temperature fields are measured using thermometers, thermocouples, or infrared cameras. These instruments detect changes in thermal properties, such as volume, resistance, or emitted infrared radiation, to determine the temperature at different points. The choice of instrument depends on the temperature range, accuracy requirements, and the nature of the system under investigation.
Applications: A Wide Spectrum
Both electric fields and temperature fields find applications in numerous scientific and engineering domains. Electric fields are integral to electronics, electromagnetism, and particle physics. Their applications range from powering our devices to accelerating particles in high-energy physics experiments.
Temperature fields are vital in thermodynamics, materials science, and meteorology. Their applications encompass everything from designing efficient heat exchangers to forecasting weather patterns.
Overlapping Applications: Thermoelectric Effects
It is worth noting that some applications involve both fields simultaneously. For example, thermoelectric effects utilize the relationship between temperature gradients and electric fields. These effects allow for the direct conversion of heat energy into electrical energy (Seebeck effect) or vice versa (Peltier effect). This demonstrates the interconnectedness of these fields, although their underlying principles remain distinct.
Conclusion: Distinct but Interrelated Fields
Electric fields and temperature fields, while both representing spatial distributions of physical quantities, are fundamentally different. Electric fields are vector fields describing forces on charges, governed by Maxwell's equations and material permittivity. Temperature fields are scalar fields describing average kinetic energy, governed by the heat equation and material thermal properties. Their interactions with matter lead to entirely different effects, with electric fields exerting forces and temperature fields driving heat transfer. While distinct, the interplay between these fields in certain phenomena highlights the rich complexity of physical processes. Understanding their differences is crucial for tackling various scientific and engineering challenges across a multitude of disciplines.
Latest Posts
Latest Posts
-
Draw The Expected Major Product Of The Reaction Shown
Apr 18, 2025
-
An Element In Period 5 And Group 3a
Apr 18, 2025
-
About 70 Of The Co2 That Diffuses Into Systemic Capillaries
Apr 18, 2025
-
Companies That Strive For Search Engine Optimization Are Looking To
Apr 18, 2025
-
When It Comes To Conflict Managers Should Understand That
Apr 18, 2025
Related Post
Thank you for visiting our website which covers about How Are Electric Field And Temperature Field Different . We hope the information provided has been useful to you. Feel free to contact us if you have any questions or need further assistance. See you next time and don't miss to bookmark.