Which Of The Following Can Serve As A Nucleophile
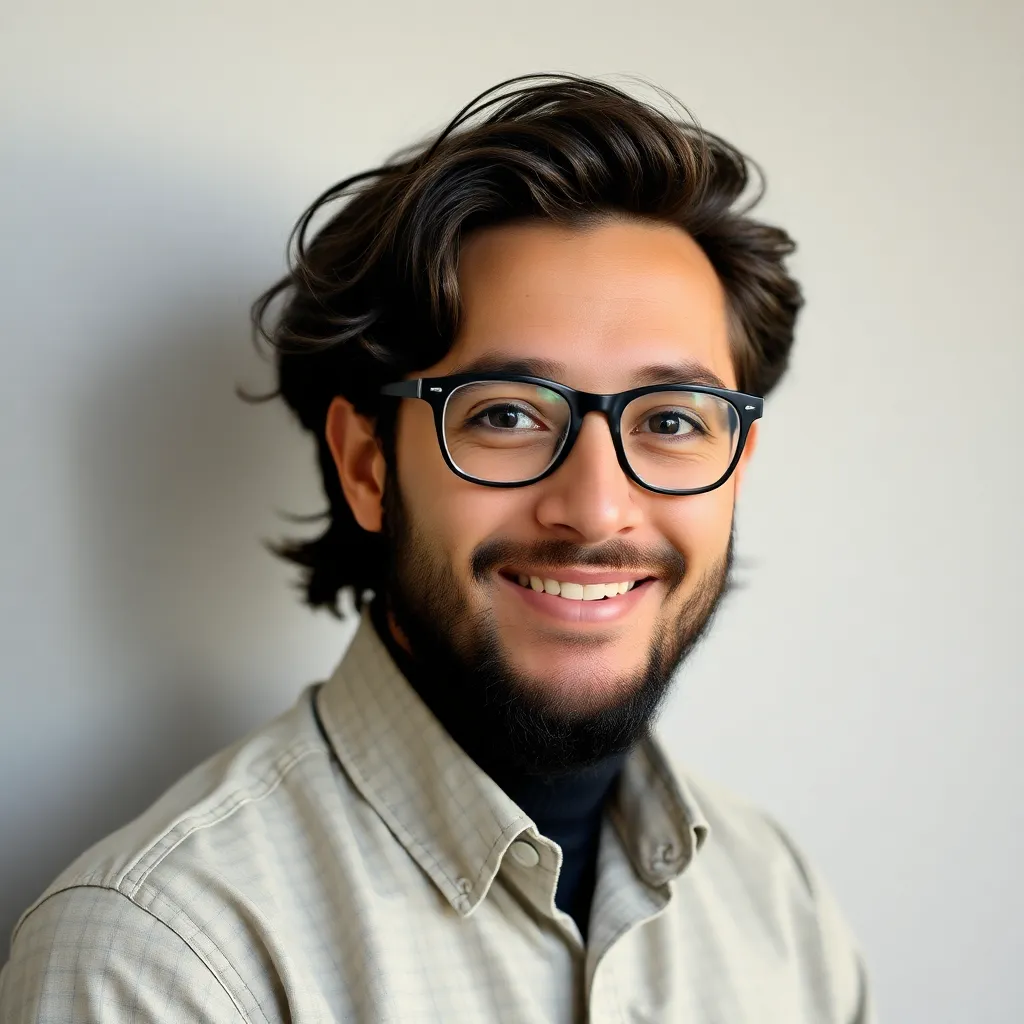
Holbox
May 11, 2025 · 6 min read
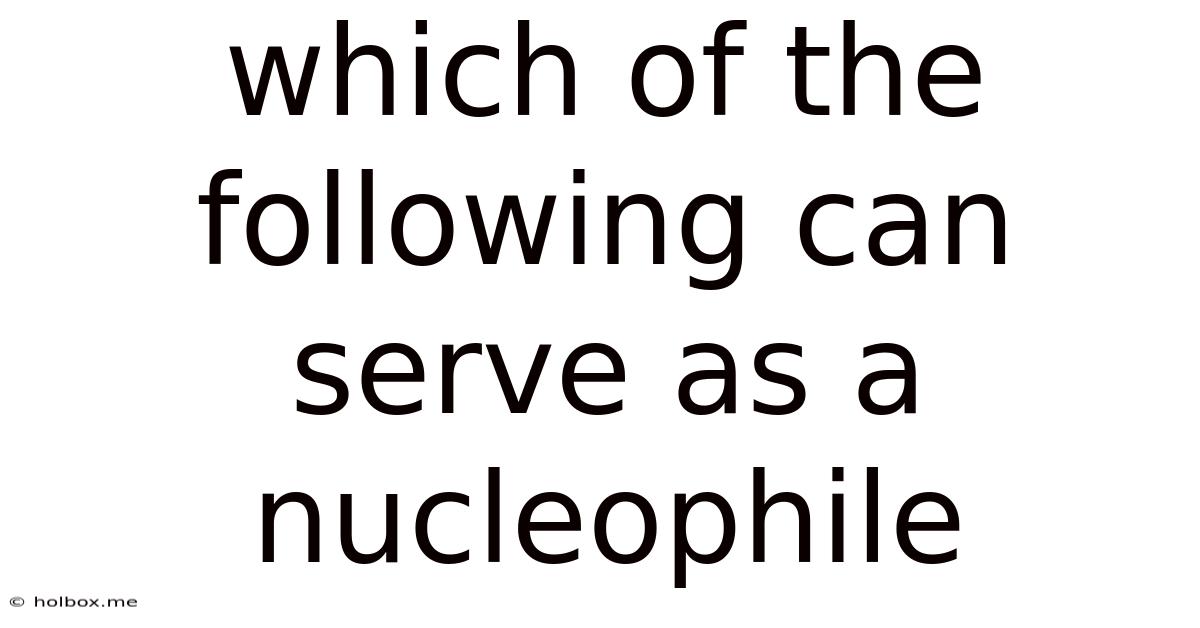
Table of Contents
- Which Of The Following Can Serve As A Nucleophile
- Table of Contents
- Which of the Following Can Serve as a Nucleophile? Understanding Nucleophilic Attack
- What is a Nucleophile?
- Factors Influencing Nucleophilicity
- 1. Charge
- 2. Electronegativity
- 3. Steric Hindrance
- 4. Solvent Effects
- 5. Resonance
- 6. Atom Type
- Identifying Potential Nucleophiles: Examples
- 1. Anions
- 2. Neutral Molecules with Lone Pairs
- 3. π-Bonds
- 4. Organometallic Reagents
- Predicting Nucleophilic Behavior: A Practical Approach
- Nucleophiles in Action: Real-World Applications
- Conclusion: Mastering Nucleophilicity
- Latest Posts
- Related Post
Which of the Following Can Serve as a Nucleophile? Understanding Nucleophilic Attack
Nucleophiles are fundamental players in organic chemistry, driving countless reactions. Understanding what makes a molecule a good nucleophile is crucial for predicting reaction outcomes and designing synthetic strategies. This article delves deep into the concept of nucleophilicity, exploring various factors that influence it and providing a comprehensive guide to identifying potential nucleophiles.
What is a Nucleophile?
A nucleophile (literally "nucleus-loving") is a chemical species that donates an electron pair to an electrophile (an electron-deficient species) to form a chemical bond. This donation occurs through a process called a nucleophilic attack. The key characteristic of a nucleophile is the presence of a lone pair of electrons or a π bond that can be donated. This electron pair is often associated with a negatively charged atom or an atom with a high electron density.
Factors Influencing Nucleophilicity
Several factors determine the strength of a nucleophile. These factors are intricately intertwined, and predicting relative nucleophilicity often requires considering multiple aspects simultaneously.
1. Charge
Negatively charged species are generally stronger nucleophiles than neutral species. The negative charge increases the electron density, making the nucleophile more readily available to donate its electrons. For instance, hydroxide ion (OH⁻) is a much stronger nucleophile than water (H₂O).
2. Electronegativity
Electronegativity is the ability of an atom to attract electrons towards itself. Less electronegative atoms make better nucleophiles. This is because less electronegative atoms hold their electrons less tightly, making them more readily available for donation. For example, sulfur (S) is a better nucleophile than oxygen (O) because sulfur is less electronegative.
3. Steric Hindrance
The size and shape of the molecule, often referred to as steric hindrance, significantly affect nucleophilicity. Bulky groups surrounding the nucleophilic atom can hinder its approach to the electrophile, reducing its effectiveness. Smaller nucleophiles generally react faster than larger ones. Compare the nucleophilicity of CH₃O⁻ (methoxide) and tert-Butoxide ((CH₃)₃CO⁻): methoxide is a much better nucleophile due to significantly less steric hindrance.
4. Solvent Effects
The solvent plays a critical role in influencing nucleophilicity. Protic solvents (solvents with O-H or N-H bonds, such as water and alcohols) can solvate (surround and stabilize) nucleophiles through hydrogen bonding. This solvation reduces the nucleophile's reactivity. In contrast, aprotic solvents (solvents lacking O-H or N-H bonds, such as DMF and DMSO) do not solvate nucleophiles as strongly, leading to increased nucleophilicity.
Therefore, the same nucleophile can exhibit different reactivities in different solvents. For instance, fluoride (F⁻) is a weak nucleophile in protic solvents but a strong nucleophile in aprotic solvents.
5. Resonance
Resonance affects nucleophilicity by delocalizing the electron density. If the nucleophile's lone pair is involved in resonance, the electron density is spread out, making it less available for donation. Thus, nucleophiles with less resonance stabilization are generally stronger nucleophiles.
6. Atom Type
The nucleophilic atom itself plays a significant role. Within the same period of the periodic table, nucleophilicity generally increases down a group. This is due to the increase in size and decrease in electronegativity down a group. For example, the order of nucleophilicity for halides is I⁻ > Br⁻ > Cl⁻ > F⁻.
Identifying Potential Nucleophiles: Examples
Let's consider several types of molecules and analyze their potential to act as nucleophiles:
1. Anions
Anions, bearing a negative charge, are inherently strong nucleophiles. Examples include:
- Hydroxide ion (OH⁻): A strong nucleophile, particularly in aprotic solvents.
- Halide ions (F⁻, Cl⁻, Br⁻, I⁻): Their nucleophilicity increases down the group (I⁻ being the strongest).
- Alkoxide ions (RO⁻): The strength of alkoxide nucleophiles depends on the size of the alkyl group (R).
- Thiolate ions (RS⁻): Sulfur is less electronegative than oxygen, making thiolates stronger nucleophiles than alkoxides.
- Carbanions (R⁻): Highly reactive nucleophiles often stabilized by resonance or electronegative groups.
2. Neutral Molecules with Lone Pairs
Neutral molecules with lone pairs of electrons can also act as nucleophiles, though generally weaker than anions. Examples include:
- Water (H₂O): A weak nucleophile.
- Ammonia (NH₃): A relatively stronger nucleophile than water.
- Alcohols (ROH): Weak nucleophiles.
- Amines (RNH₂, R₂NH, R₃N): Nucleophilicity increases with the number of alkyl groups (R).
- Thiols (RSH): Stronger nucleophiles than alcohols due to the less electronegative sulfur.
3. π-Bonds
Molecules with π-bonds can act as nucleophiles. The π electrons can be donated to an electrophile, leading to the formation of a new σ bond. Examples include:
- Alkenes: React with electrophiles through electrophilic addition reactions.
- Alkynes: Similar to alkenes, but generally more reactive due to the higher electron density in the triple bond.
- Arenes: Though less reactive than alkenes and alkynes, arenes can undergo electrophilic aromatic substitution reactions.
4. Organometallic Reagents
Organometallic reagents, such as Grignard reagents (RMgX) and organolithium reagents (RLi), are exceptionally strong nucleophiles due to the highly polarized carbon-metal bond. The carbon atom bears a significant partial negative charge, making it a powerful nucleophile.
Predicting Nucleophilic Behavior: A Practical Approach
To determine whether a molecule can serve as a nucleophile, systematically consider the factors discussed above:
- Identify potential nucleophilic centers: Look for atoms with lone pairs or π-bonds.
- Assess the charge: Negatively charged species are stronger nucleophiles.
- Consider electronegativity: Less electronegative atoms are better nucleophiles.
- Evaluate steric hindrance: Bulky groups reduce nucleophilicity.
- Account for solvent effects: Protic solvents weaken nucleophiles, while aprotic solvents enhance them.
- Analyze resonance: Delocalization of electron density reduces nucleophilicity.
- Compare atom types: Nucleophilicity increases down a group in the periodic table.
By carefully analyzing these factors, you can effectively predict whether a molecule will act as a nucleophile and assess its relative reactivity. This understanding is crucial for success in organic chemistry, allowing for the design and prediction of various chemical reactions.
Nucleophiles in Action: Real-World Applications
Nucleophilic reactions are ubiquitous in chemistry and have widespread applications in various fields:
-
Organic Synthesis: Nucleophilic substitution and addition reactions are fundamental tools for building complex organic molecules. Drugs, polymers, and other materials are synthesized using these reactions.
-
Biochemistry: Many biochemical processes, such as enzyme catalysis and DNA replication, involve nucleophilic attacks. Nucleophiles play a crucial role in the function of many biological molecules.
-
Materials Science: Nucleophilic reactions are used in the synthesis of new materials with specific properties, such as polymers, coatings, and semiconductors.
-
Industrial Processes: Many industrial processes rely on nucleophilic reactions, such as the production of detergents, plastics, and pharmaceuticals.
Conclusion: Mastering Nucleophilicity
Understanding nucleophilicity is essential for any serious student or practitioner of organic chemistry. By considering the interplay of charge, electronegativity, steric hindrance, solvent effects, resonance, and atom type, one can effectively predict and manipulate the reactivity of nucleophiles. This knowledge is not only vital for understanding fundamental chemical reactions but also for developing new synthetic routes and designing innovative materials with desired properties. The power of nucleophilic chemistry extends far beyond the classroom, impacting various sectors of science and industry. This comprehensive understanding of nucleophilic reactions opens doors to a deeper comprehension of the chemical world and its transformative potential.
Latest Posts
Related Post
Thank you for visiting our website which covers about Which Of The Following Can Serve As A Nucleophile . We hope the information provided has been useful to you. Feel free to contact us if you have any questions or need further assistance. See you next time and don't miss to bookmark.