What Reagent Is Required To Accomplish The Following Transformation
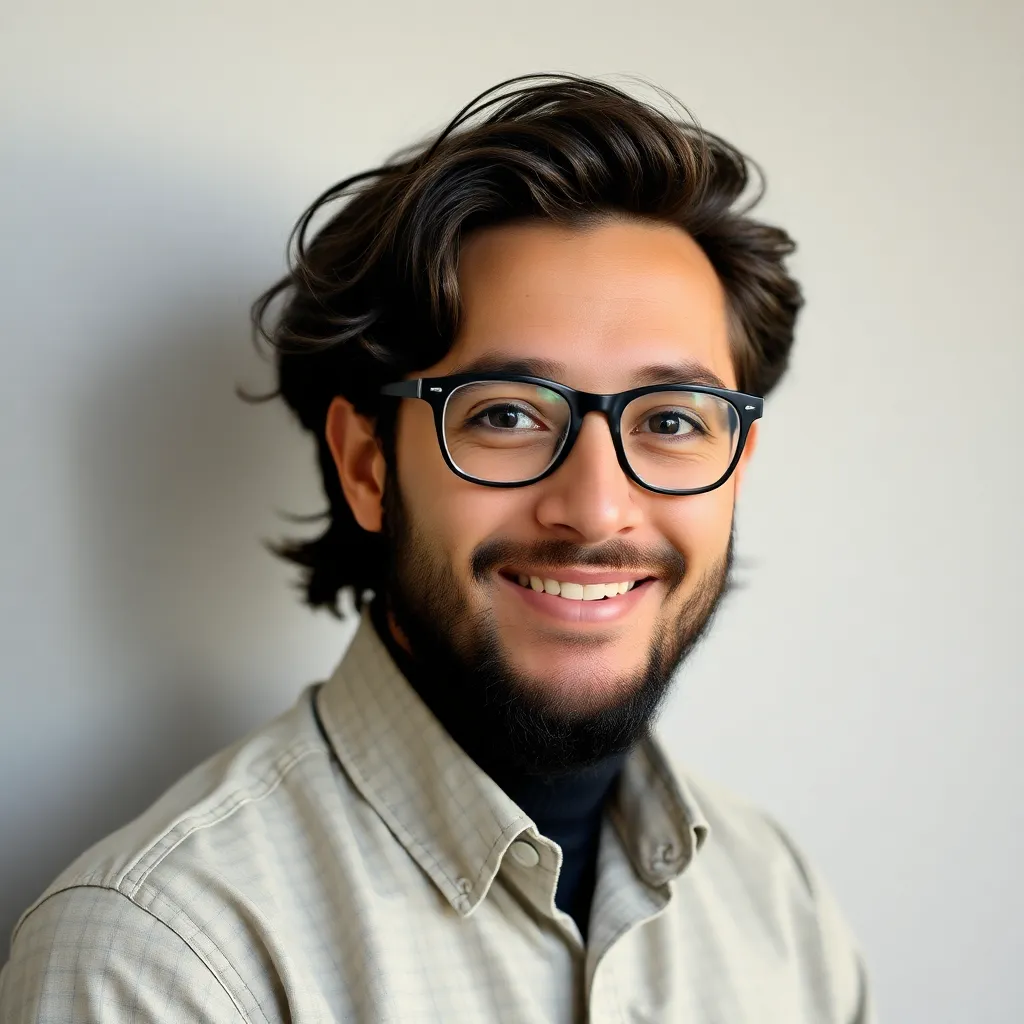
Holbox
May 08, 2025 · 6 min read
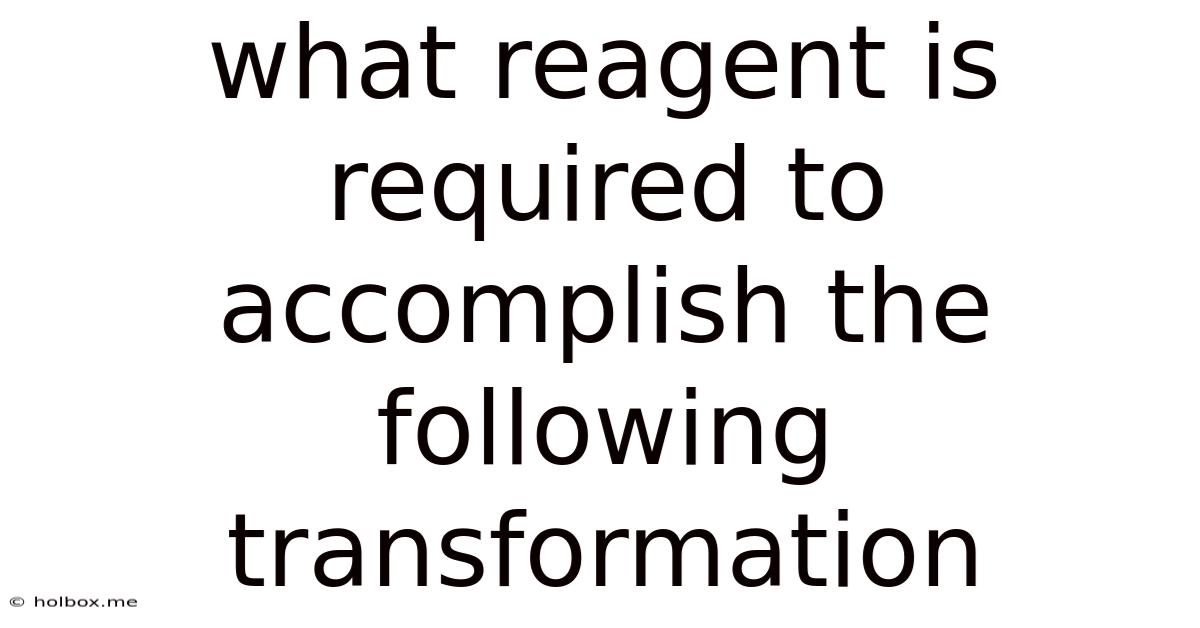
Table of Contents
- What Reagent Is Required To Accomplish The Following Transformation
- Table of Contents
- What Reagent is Required to Accomplish the Following Transformation? A Comprehensive Guide
- Understanding the Basics: Functional Group Transformations
- Key Factors Influencing Reagent Selection
- Examples of Common Transformations and Required Reagents
- 1. Oxidation Reactions
- 2. Reduction Reactions
- 3. Substitution Reactions
- 4. Addition Reactions
- 5. Elimination Reactions
- Advanced Transformations and Reagent Considerations
- Importance of Understanding Reaction Mechanisms
- Conclusion: A Continuous Learning Process
- Latest Posts
- Related Post
What Reagent is Required to Accomplish the Following Transformation? A Comprehensive Guide
Determining the necessary reagent for a specific chemical transformation is a cornerstone of organic chemistry. This seemingly simple question—"What reagent is required?"—often unlocks complex synthetic pathways and requires a deep understanding of reaction mechanisms and functional group reactivity. This article will delve into this crucial aspect of organic synthesis, exploring various transformation types and the reagents commonly employed to achieve them. We'll cover a range of reactions, from simple functional group interconversions to more complex multi-step processes. Remember, always consult relevant literature and safety data sheets before attempting any chemical reaction.
Understanding the Basics: Functional Group Transformations
Before diving into specific examples, it's vital to understand the fundamental concept of functional group transformations. A functional group is an atom or group of atoms within a molecule that is responsible for its characteristic chemical reactions. Transforming one functional group into another is often the primary goal of organic synthesis. The choice of reagent is directly dictated by the desired transformation and the starting material's structure.
Key Factors Influencing Reagent Selection
Several crucial factors influence the selection of the appropriate reagent:
- Starting Material: The nature of the starting material's functional groups significantly impacts the choice of reagent. A reagent compatible with one functional group might be detrimental to another present in the molecule.
- Desired Product: The target molecule and its functional groups determine the necessary transformation. This dictates the type of reaction (e.g., oxidation, reduction, substitution, addition, elimination).
- Reaction Conditions: Factors like temperature, solvent, and pH significantly influence reaction efficiency and selectivity. Some reagents require specific conditions for optimal performance.
- Selectivity: Ideally, a reagent should be selective, reacting primarily with the desired functional group while leaving others untouched. This minimizes side reactions and improves yield.
- Cost and Availability: Practical considerations, such as reagent cost and availability, also play a role in the selection process.
Examples of Common Transformations and Required Reagents
Let's explore several common transformations and the reagents used to accomplish them. These examples illustrate the principles discussed above and demonstrate the diverse range of reagents available to synthetic chemists.
1. Oxidation Reactions
Oxidation reactions involve the loss of electrons by a molecule, often accompanied by an increase in the oxidation state of an atom. Common oxidations include:
-
Alcohols to Aldehydes/Ketones: Several reagents can oxidize alcohols, with the choice depending on the desired level of oxidation (aldehyde vs. ketone) and the sensitivity of other functional groups. PCC (pyridinium chlorochromate) is a mild oxidant suitable for converting primary alcohols to aldehydes. Jones reagent (chromic acid) is a stronger oxidant that typically converts primary alcohols to carboxylic acids and secondary alcohols to ketones. Swern oxidation (using DMSO, oxalyl chloride, and a base) is another widely used method for oxidizing alcohols to aldehydes or ketones, offering good selectivity.
-
Aldehydes to Carboxylic Acids: Stronger oxidizing agents are required to convert aldehydes to carboxylic acids. Potassium permanganate (KMnO4) and Jones reagent are commonly employed for this transformation.
2. Reduction Reactions
Reduction reactions involve the gain of electrons by a molecule, often resulting in a decrease in the oxidation state of an atom. Common reductions include:
-
Ketones/Aldehydes to Alcohols: Sodium borohydride (NaBH4) is a mild reducing agent suitable for reducing ketones and aldehydes to alcohols. Lithium aluminum hydride (LiAlH4) is a more powerful reducing agent capable of reducing a wider range of functional groups, including esters and carboxylic acids.
-
Alkynes to Alkenes/Alkanes: Hydrogenation, using a metal catalyst like platinum (Pt), palladium (Pd), or nickel (Ni), can reduce alkynes to alkenes or alkanes depending on the catalyst and reaction conditions. Lindlar catalyst (Pd/CaCO3 poisoned with lead acetate and quinoline) is a selective catalyst that reduces alkynes to cis-alkenes.
3. Substitution Reactions
Substitution reactions involve the replacement of one atom or group with another. Examples include:
-
SN1 and SN2 Reactions: The type of substitution reaction (SN1 or SN2) depends on the substrate, nucleophile, and solvent. SN2 reactions often employ strong nucleophiles like sodium cyanide (NaCN), sodium azide (NaN3), or sodium hydroxide (NaOH). SN1 reactions typically involve weaker nucleophiles and often proceed through a carbocation intermediate.
-
Electrophilic Aromatic Substitution: These reactions involve the replacement of a hydrogen atom on an aromatic ring with an electrophile. Common electrophiles include bromine (Br2), nitric acid (HNO3), and sulfuric acid (H2SO4). Lewis acids like aluminum chloride (AlCl3) are often used as catalysts.
4. Addition Reactions
Addition reactions involve the addition of atoms or groups to a multiple bond (e.g., alkene or alkyne). Examples include:
-
Addition of HBr to Alkenes: Hydrogen bromide (HBr) adds across the double bond of an alkene, following Markovnikov's rule (the hydrogen atom adds to the carbon atom with more hydrogen atoms).
-
Hydroboration-Oxidation: This reaction sequence adds a hydroxyl group across the double bond of an alkene in an anti-Markovnikov fashion. Borane (BH3) is used in the hydroboration step, followed by oxidation with hydrogen peroxide (H2O2) and a base.
5. Elimination Reactions
Elimination reactions involve the removal of atoms or groups from a molecule, often resulting in the formation of a multiple bond. Examples include:
-
Dehydration of Alcohols: Strong acids like sulfuric acid (H2SO4) or phosphoric acid (H3PO4) can dehydrate alcohols to form alkenes.
-
Dehydrohalogenation: Strong bases like potassium hydroxide (KOH) or sodium ethoxide (NaOEt) can remove a hydrogen halide from an alkyl halide to form an alkene.
Advanced Transformations and Reagent Considerations
The examples above represent a small fraction of the numerous transformations possible in organic chemistry. Many reactions involve more complex mechanisms and require specialized reagents. Some examples include:
-
Grignard Reactions: Organomagnesium halides (Grignard reagents) are powerful nucleophiles used to form carbon-carbon bonds. They react with a variety of electrophiles, including aldehydes, ketones, esters, and epoxides.
-
Wittig Reactions: This reaction is used to convert aldehydes and ketones to alkenes. The Wittig reagent, a phosphorus ylide, reacts with the carbonyl group to form an alkene.
-
Diels-Alder Reactions: This is a [4+2] cycloaddition reaction that forms a six-membered ring from a diene and a dienophile.
-
Suzuki Coupling: This palladium-catalyzed cross-coupling reaction is used to form carbon-carbon bonds between an aryl or vinyl halide and an organoboron compound.
-
Heck Reaction: Another palladium-catalyzed reaction that forms carbon-carbon bonds between an aryl or vinyl halide and an alkene.
Importance of Understanding Reaction Mechanisms
Understanding the underlying reaction mechanism is crucial for choosing the appropriate reagent. The mechanism dictates the reactivity of the functional groups and the stereochemistry of the product. For example, understanding the SN1 and SN2 mechanisms allows for a rational choice of reagent based on the substrate and desired stereochemistry.
Conclusion: A Continuous Learning Process
Determining the necessary reagent to achieve a specific transformation is a complex but rewarding aspect of organic chemistry. It demands a thorough understanding of reaction mechanisms, functional group reactivity, and various reagent properties. This article has provided a broad overview of common transformations and the associated reagents, but it is crucial to remember that this is a continually evolving field. Consistent learning, consultation of relevant literature, and careful experimental design are essential for successful synthesis. Always prioritize safety and consult safety data sheets before working with any chemicals. With dedication and a systematic approach, mastering the art of reagent selection becomes a cornerstone of successful organic synthesis.
Latest Posts
Related Post
Thank you for visiting our website which covers about What Reagent Is Required To Accomplish The Following Transformation . We hope the information provided has been useful to you. Feel free to contact us if you have any questions or need further assistance. See you next time and don't miss to bookmark.