Undergoes Alpha Decay Forming An Alpha Particle And
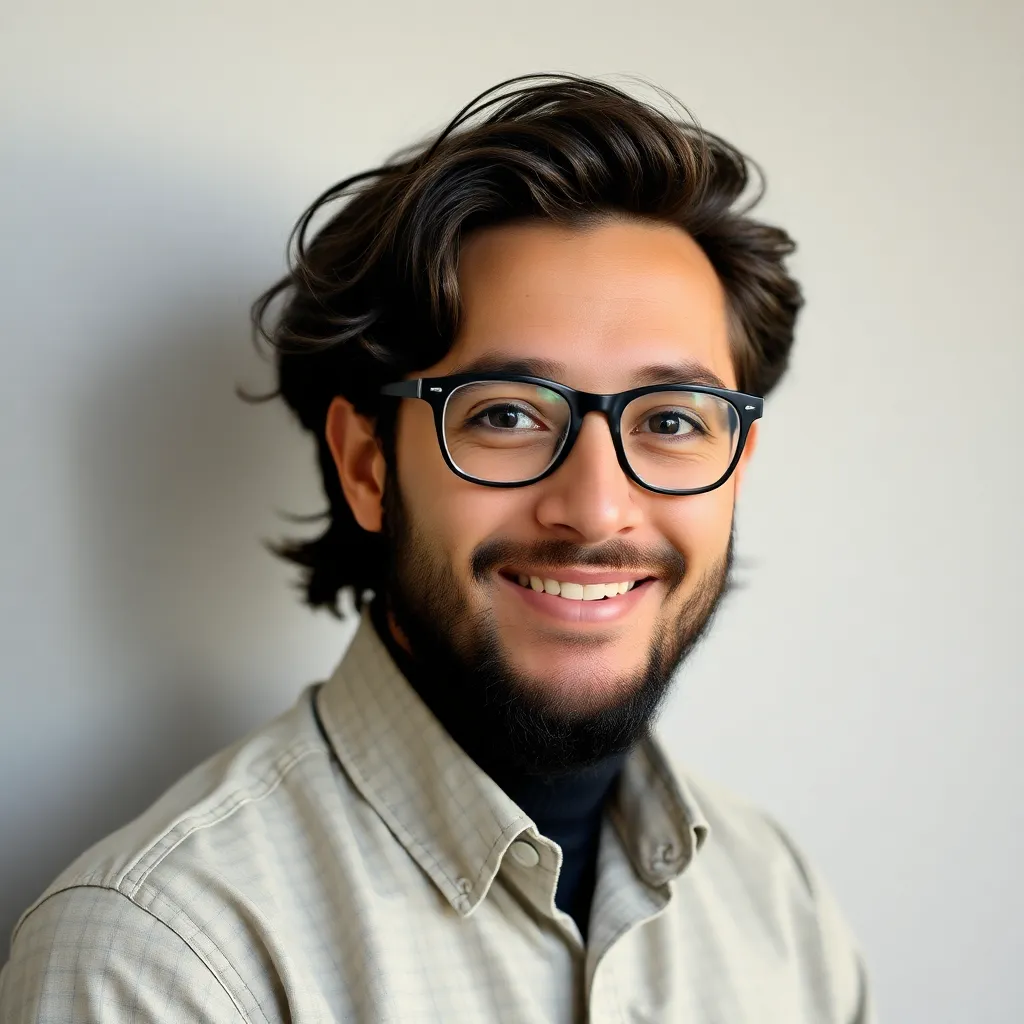
Holbox
May 13, 2025 · 7 min read
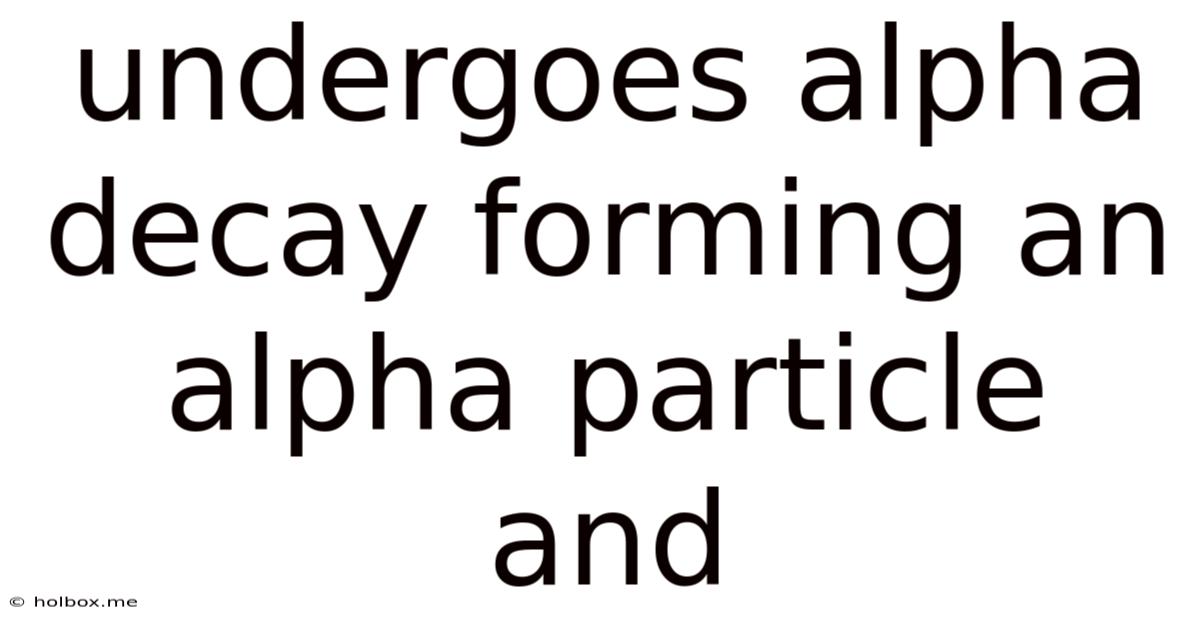
Table of Contents
- Undergoes Alpha Decay Forming An Alpha Particle And
- Table of Contents
- Undergoes Alpha Decay Forming an Alpha Particle and… What Happens Next?
- What is Alpha Decay?
- The Mechanics of Alpha Decay: Quantum Tunneling
- The Daughter Nucleus: Properties and Characteristics
- Factors Affecting Alpha Decay Rate
- Alpha Decay and the Chart of Nuclides
- Applications and Significance of Alpha Decay
- Beyond the Alpha Particle: Subsequent Decays and Decay Chains
- Detecting Alpha Decay
- Conclusion: A Cornerstone of Nuclear Physics
- Latest Posts
- Latest Posts
- Related Post
Undergoes Alpha Decay Forming an Alpha Particle and… What Happens Next?
Alpha decay, a fundamental process in nuclear physics, plays a crucial role in the stability and transformation of atomic nuclei. This process involves the emission of an alpha particle, effectively altering the composition and properties of the parent nucleus. Understanding alpha decay requires delving into the intricacies of nuclear structure, quantum mechanics, and the forces that govern the subatomic world. This comprehensive exploration will dissect the phenomenon of alpha decay, examining the mechanisms involved, the resulting daughter nuclei, and the broader implications within nuclear physics and beyond.
What is Alpha Decay?
Alpha decay is a type of radioactive decay where an unstable atomic nucleus emits an alpha particle, transforming into a new nucleus with a lower atomic number and mass number. An alpha particle (α) is essentially a helium-4 nucleus, consisting of two protons and two neutrons, represented as ⁴He²⁺. This ejection signifies a significant change in the parent nucleus's composition and energy state. The process is governed by the strong nuclear force, which binds protons and neutrons together within the nucleus, and the electromagnetic force, which repels the positively charged protons.
The instability leading to alpha decay arises from an imbalance between these forces. In heavy nuclei with a high proton-to-neutron ratio, the electrostatic repulsion between protons can overcome the strong nuclear force, making the nucleus energetically unstable. Alpha decay serves as a mechanism to reduce this instability, leading to a more stable configuration.
The Mechanics of Alpha Decay: Quantum Tunneling
The emission of an alpha particle isn't a simple ejection; it's a complex quantum mechanical process involving quantum tunneling. The alpha particle, even though it possesses sufficient energy to escape the nucleus, is initially confined within the nuclear potential well, a region defined by the strong nuclear force. Classically, the alpha particle wouldn't have enough energy to overcome the potential barrier and escape.
However, the principles of quantum mechanics allow the alpha particle to "tunnel" through this potential barrier. This means there's a non-zero probability that the alpha particle can penetrate the barrier and escape, even though it lacks the classical energy to do so. The probability of tunneling is heavily dependent on the height and width of the potential barrier, which are determined by the specific nucleus undergoing decay. Nuclei with higher potential barriers exhibit slower decay rates, leading to longer half-lives.
The Daughter Nucleus: Properties and Characteristics
After undergoing alpha decay, the parent nucleus transforms into a daughter nucleus. This daughter nucleus has an atomic number two units less and a mass number four units less than the parent nucleus. For instance, if Uranium-238 (²³⁸U₉₂), a common isotope found in nature, undergoes alpha decay, it transforms into Thorium-234 (²³⁴Th₉₀).
The properties of the daughter nucleus are significantly different from the parent nucleus. This change includes its:
- Atomic Number (Z): Reduced by 2, reflecting the loss of two protons.
- Mass Number (A): Reduced by 4, reflecting the loss of two protons and two neutrons.
- Nuclear Stability: Often, but not always, closer to the "valley of stability" on a chart of nuclides. The valley of stability represents the region where nuclei are most stable.
- Radioactivity: The daughter nucleus itself might be radioactive, potentially undergoing further decay through alpha, beta, or gamma decay.
Understanding these changes is critical in predicting the decay chain of a radioactive isotope. Many heavy radioactive isotopes undergo a series of alpha decays, eventually leading to a stable isotope. These decay chains are essential in various applications, including nuclear dating and radiation detection.
Factors Affecting Alpha Decay Rate
The rate at which alpha decay occurs, characterized by the half-life, varies significantly among different nuclei. Several factors influence this decay rate:
- Nuclear Size and Shape: Larger nuclei with a greater number of protons and neutrons generally have a higher probability of alpha decay. The shape of the nucleus also plays a role, with deformed nuclei exhibiting altered decay rates.
- Potential Barrier Height and Width: As previously mentioned, the height and width of the potential barrier directly impact the tunneling probability and, consequently, the decay rate. Higher and wider barriers result in slower decay rates.
- Coulomb Repulsion: The electrostatic repulsion between the positively charged alpha particle and the remaining daughter nucleus influences the decay rate. Stronger repulsion leads to a slower decay rate.
- Nuclear Structure: The specific arrangement of protons and neutrons within the nucleus affects the probability of alpha particle formation and its subsequent tunneling. Certain nuclear configurations favor alpha decay over other decay modes.
Alpha Decay and the Chart of Nuclides
The chart of nuclides, a graphical representation of all known isotopes, provides valuable insights into alpha decay. It plots isotopes based on their neutron and proton numbers. Alpha decay is represented by a diagonal line with a slope of 2/2 = 1, since both the proton and neutron number decrease by two respectively. Following the decay path on the chart of nuclides allows us to predict the series of decays and the eventual stable product.
Applications and Significance of Alpha Decay
Alpha decay, though seemingly a fundamental nuclear process, has significant applications and implications across various fields:
- Nuclear Dating: Alpha decay rates are used in radiometric dating techniques, such as uranium-lead dating, to determine the age of geological samples and artifacts. The constant decay rate of specific isotopes allows scientists to estimate the time elapsed since the sample's formation.
- Radiation Detection: Alpha particles, due to their relatively high ionizing power, are easily detectable using various radiation detectors. This property is crucial in radiation monitoring and safety applications.
- Nuclear Medicine: Alpha-emitting isotopes have shown promise in targeted alpha therapy, a cancer treatment approach where alpha particles are directed towards cancerous cells, minimizing damage to healthy tissues.
- Nuclear Energy: Though less prominent compared to fission, alpha decay plays a role in the energy production of certain radioactive materials, contributing to the heat generated in nuclear reactors and radioactive waste.
- Understanding Stellar Nucleosynthesis: Alpha decay is a key process in understanding the formation of elements within stars. It plays a vital role in the synthesis of heavier elements from lighter ones.
Beyond the Alpha Particle: Subsequent Decays and Decay Chains
It is important to note that the daughter nucleus formed after alpha decay is not always stable. Often, the daughter nucleus is itself radioactive and undergoes further decay, leading to a decay chain. These chains can consist of multiple steps involving alpha, beta, or gamma decay, eventually culminating in a stable isotope. Understanding these decay chains is critical for predicting the long-term behavior of radioactive materials and managing nuclear waste. The decay chain of Uranium-238, for example, involves a series of alpha and beta decays, ultimately leading to stable Lead-206.
Detecting Alpha Decay
Alpha particles, due to their relatively large mass and charge, interact strongly with matter. This strong interaction leads to a high rate of ionization as they pass through a medium. This property is exploited in various detection methods:
- Gas-filled detectors: Alpha particles ionize gas molecules within a detector, creating an electrical signal that can be measured.
- Scintillation detectors: Alpha particles excite atoms in a scintillation material, causing it to emit light. The light is then detected by a photomultiplier tube.
- Solid-state detectors: These detectors utilize the ionization of silicon or other semiconductor materials to produce an electrical signal.
These techniques allow for precise measurements of alpha particle energy and flux, providing valuable insights into the decay process.
Conclusion: A Cornerstone of Nuclear Physics
Alpha decay, a fundamental process in nuclear physics, has far-reaching implications. From the intricacies of quantum tunneling to its crucial role in stellar nucleosynthesis and radioactive dating, its understanding is essential for advancements in various scientific and technological fields. Its impact continues to be relevant in nuclear medicine, energy production, and the management of radioactive waste. Continued research and exploration of alpha decay promise to unveil further insights into the fascinating world of nuclear physics and its implications for our understanding of the universe.
Latest Posts
Latest Posts
-
How Many Hours In 3 Weeks
May 19, 2025
-
How Many Kg In 165 Pounds
May 19, 2025
-
How Tall Is 153cm In Feet
May 19, 2025
-
What Is 175 Pounds In Kg
May 19, 2025
-
How Many Cups In 650 Ml
May 19, 2025
Related Post
Thank you for visiting our website which covers about Undergoes Alpha Decay Forming An Alpha Particle And . We hope the information provided has been useful to you. Feel free to contact us if you have any questions or need further assistance. See you next time and don't miss to bookmark.