This Contractile Protein Forms The Thin Filaments
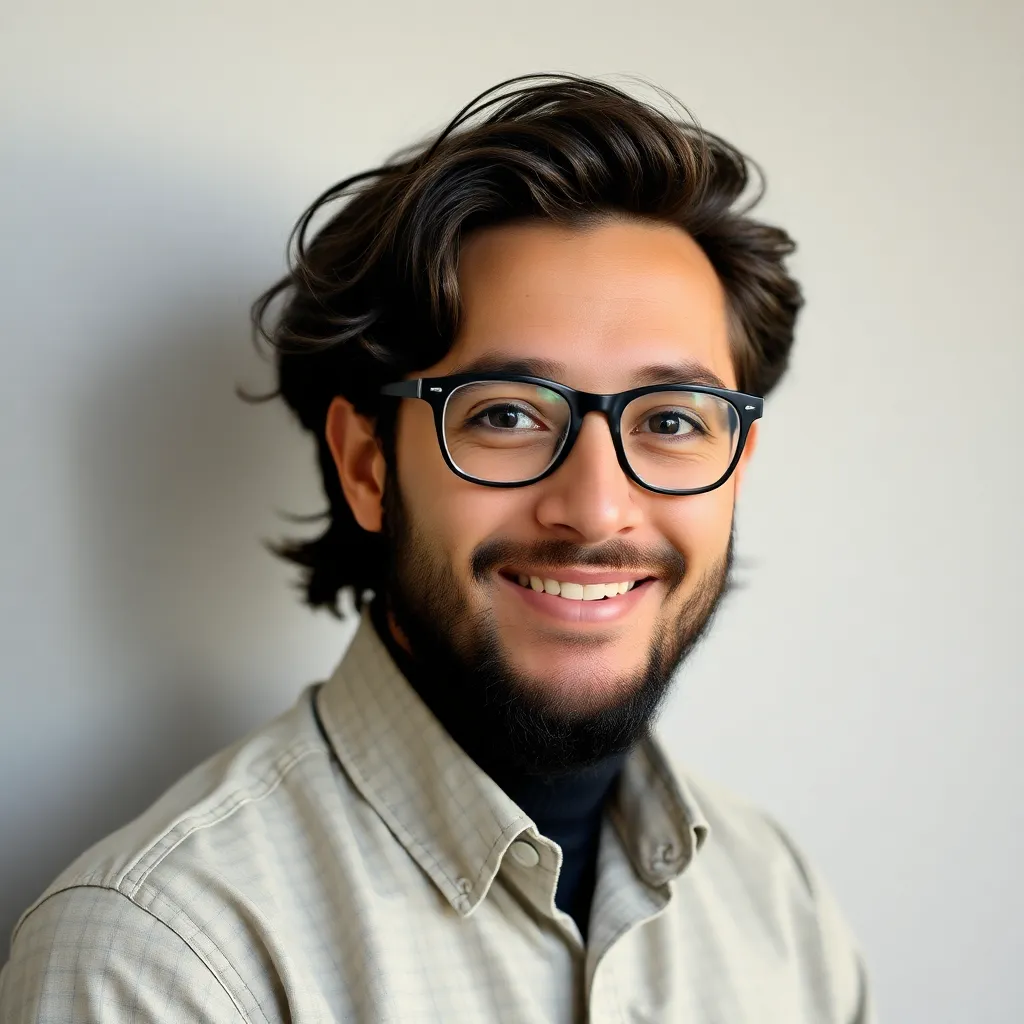
Holbox
Apr 08, 2025 · 6 min read

Table of Contents
- This Contractile Protein Forms The Thin Filaments
- Table of Contents
- This Contractile Protein Forms the Thin Filaments: A Deep Dive into Actin
- The Structure of Actin: A Molecular Marvel
- From G-actin to F-actin: Polymerization and Dynamics
- Actin-Binding Proteins: Orchestrating Actin's Function
- Key Actin-Binding Proteins and Their Roles:
- Actin's Role in Muscle Contraction: The Sarcomere's Dynamic Duo
- The Sliding Filament Theory: A Symphony of Actin and Myosin
- Actin's Diverse Roles Beyond Muscle Contraction
- Cell Motility: Crawling and Reaching
- Cytokinesis: Dividing Cells
- Vesicle Transport: Intracellular Highways
- Cell Shape and Structure: Maintaining Integrity
- Signal Transduction: Communicating Networks
- Clinical Significance of Actin Dysfunction
- Conclusion: Actin – A Master Regulator of Cellular Processes
- Latest Posts
- Latest Posts
- Related Post
This Contractile Protein Forms the Thin Filaments: A Deep Dive into Actin
Actin, the answer to the question posed in the title, is a crucial contractile protein forming the thin filaments in muscle cells. Understanding actin's structure, function, and interactions within the muscle sarcomere is key to grasping the mechanics of muscle contraction. This article will delve deep into the world of actin, exploring its multifaceted roles and significance in various biological processes.
The Structure of Actin: A Molecular Marvel
Actin exists in two primary forms: globular actin (G-actin) and filamentous actin (F-actin). G-actin is a monomeric protein, a single polypeptide chain folded into a compact globular structure. It possesses a nucleotide-binding site, typically occupied by ATP (adenosine triphosphate) or ADP (adenosine diphosphate), which plays a crucial role in its polymerization and function. The ATP hydrolysis cycle is intrinsically linked to actin's dynamic properties.
From G-actin to F-actin: Polymerization and Dynamics
G-actin monomers polymerize to form F-actin, a double-helical structure. This polymerization process is tightly regulated and involves several key steps:
-
Nucleation: The initial step involves the formation of a stable trimer or tetramer of G-actin, which acts as a nucleus for further polymerization. This step is often the rate-limiting step in the process.
-
Elongation: Once the nucleus is formed, G-actin monomers add to both ends of the growing filament. The rate of addition is faster at the "barbed" or plus (+) end compared to the "pointed" or minus (-) end.
-
Steady State: At equilibrium, the rate of addition of monomers equals the rate of dissociation, leading to a steady-state concentration of F-actin.
The dynamic nature of actin filaments is critical for their function. Treadmilling, a process where G-actin monomers add to the plus end and dissociate from the minus end, allows for rapid remodeling and adaptation to changing cellular needs. This dynamic instability is crucial for processes like cell motility and cytokinesis.
Actin-Binding Proteins: Orchestrating Actin's Function
Actin's activity isn't solely dependent on its intrinsic properties. A vast array of actin-binding proteins (ABPs) regulate its polymerization, depolymerization, bundling, branching, and interactions with other cellular components. These proteins are essential in determining the organization and function of actin filaments in various cellular contexts.
Key Actin-Binding Proteins and Their Roles:
-
Thymosin β4: This protein sequesters G-actin monomers, preventing their spontaneous polymerization. It acts as a reservoir of G-actin, ready for polymerization when needed.
-
Profilin: This protein promotes actin polymerization by facilitating the exchange of ADP for ATP on G-actin, making it more readily available for addition to the growing filament.
-
Cofilin: This protein binds to ADP-F-actin, promoting depolymerization and enhancing the turnover of actin filaments. It plays a critical role in regulating the dynamic instability of actin filaments.
-
Formins: These proteins nucleate the formation of long, unbranched actin filaments. They play important roles in cell motility and cytokinesis.
-
Arp2/3 complex: This complex nucleates the formation of branched actin networks. It is crucial for the formation of lamellipodia and filopodia, cellular structures involved in cell motility and adhesion.
-
Filamin: This protein cross-links actin filaments, forming a gel-like network. It plays a role in regulating cell shape and mechanical properties.
Actin's Role in Muscle Contraction: The Sarcomere's Dynamic Duo
In muscle cells, actin forms the thin filaments of the sarcomere, the basic contractile unit. The sarcomere consists of overlapping thin and thick filaments composed of actin and myosin, respectively. The interaction between these filaments, regulated by calcium ions and ATP, underlies the process of muscle contraction.
The Sliding Filament Theory: A Symphony of Actin and Myosin
The sliding filament theory explains muscle contraction as the sliding of thin filaments (actin) over thick filaments (myosin). This sliding is powered by the cyclical interaction between myosin heads and actin filaments.
-
Myosin Heads Bind to Actin: The myosin heads, which possess ATPase activity, bind to specific sites on actin filaments (in the presence of calcium ions).
-
Power Stroke: ATP hydrolysis triggers a conformational change in the myosin head, causing it to pivot and pull the actin filament towards the center of the sarcomere.
-
Detachment: A new ATP molecule binds to the myosin head, causing it to detach from the actin filament.
-
Re-cocking: ATP hydrolysis re-cocks the myosin head, preparing it for the next cycle.
This cycle repeats continuously, resulting in the sliding of actin filaments over myosin filaments and the shortening of the sarcomere, leading to muscle contraction.
Actin's Diverse Roles Beyond Muscle Contraction
While actin's role in muscle contraction is perhaps its most well-known function, its importance extends far beyond the musculoskeletal system. Actin filaments play crucial roles in a wide range of cellular processes:
Cell Motility: Crawling and Reaching
Actin filaments are central to cell motility, the ability of cells to move. Their dynamic organization, driven by ABPs, enables cells to extend protrusions like lamellipodia and filopodia, adhere to substrates, and generate the force needed for movement. This is crucial for processes such as wound healing, immune responses, and embryonic development.
Cytokinesis: Dividing Cells
Actin filaments play a crucial role in cytokinesis, the final stage of cell division, where the cytoplasm divides to form two daughter cells. A contractile ring, composed primarily of actin and myosin, forms around the equator of the cell and constricts, pinching the cell in two.
Vesicle Transport: Intracellular Highways
Actin filaments serve as tracks for intracellular transport, guiding the movement of vesicles and organelles within the cell. Motor proteins like myosin I move along actin filaments, carrying their cargo.
Cell Shape and Structure: Maintaining Integrity
Actin filaments contribute significantly to cell shape and mechanical stability. Their organization, regulated by ABPs, determines the cell's morphology and ability to withstand mechanical stress.
Signal Transduction: Communicating Networks
Actin filaments are also involved in signal transduction pathways, acting as platforms for the assembly and activation of signaling molecules. Their organization can influence the localization and activity of signaling components.
Clinical Significance of Actin Dysfunction
Disruptions in actin dynamics and function are linked to various diseases and disorders:
-
Muscle Diseases: Mutations in actin genes or defects in actin-binding proteins can cause various muscle disorders, including cardiomyopathies (heart muscle diseases) and muscular dystrophies.
-
Cancers: Actin dysregulation plays a role in cancer cell metastasis, the spread of cancer cells to other parts of the body. Changes in actin dynamics can affect cell motility, invasion, and angiogenesis (formation of new blood vessels).
-
Neurological Disorders: Actin dysfunction has been implicated in several neurological disorders, including Alzheimer's disease and Parkinson's disease. Disruptions in actin dynamics can affect neuronal growth, synapse formation, and synaptic plasticity.
Conclusion: Actin – A Master Regulator of Cellular Processes
Actin, the contractile protein forming the thin filaments, is a fundamental component of eukaryotic cells. Its dynamic nature, regulated by a vast array of actin-binding proteins, allows it to perform a diverse array of functions, ranging from muscle contraction to cell motility, cytokinesis, and signal transduction. Understanding the intricacies of actin's structure, function, and regulation is crucial for advancing our knowledge of cellular processes and developing therapies for various diseases. Further research into the intricacies of actin's interactions and regulatory mechanisms promises to uncover even more about its profound impact on cell biology and human health. The dynamic world of actin continues to fascinate and challenge researchers, revealing new layers of complexity and importance with every discovery.
Latest Posts
Latest Posts
-
Scientific Thinking Using Forensics To Uncover Illegal Whaling
Apr 22, 2025
-
Which Is Not Likely Sexual Harassment
Apr 22, 2025
-
A Continuous Random Variable May Assume What Value
Apr 22, 2025
-
What Would Happen If You Ate Uranium
Apr 22, 2025
-
Elementary Statistics A Step By Step Approach
Apr 22, 2025
Related Post
Thank you for visiting our website which covers about This Contractile Protein Forms The Thin Filaments . We hope the information provided has been useful to you. Feel free to contact us if you have any questions or need further assistance. See you next time and don't miss to bookmark.