The Lock And Key Mechanism Refers To
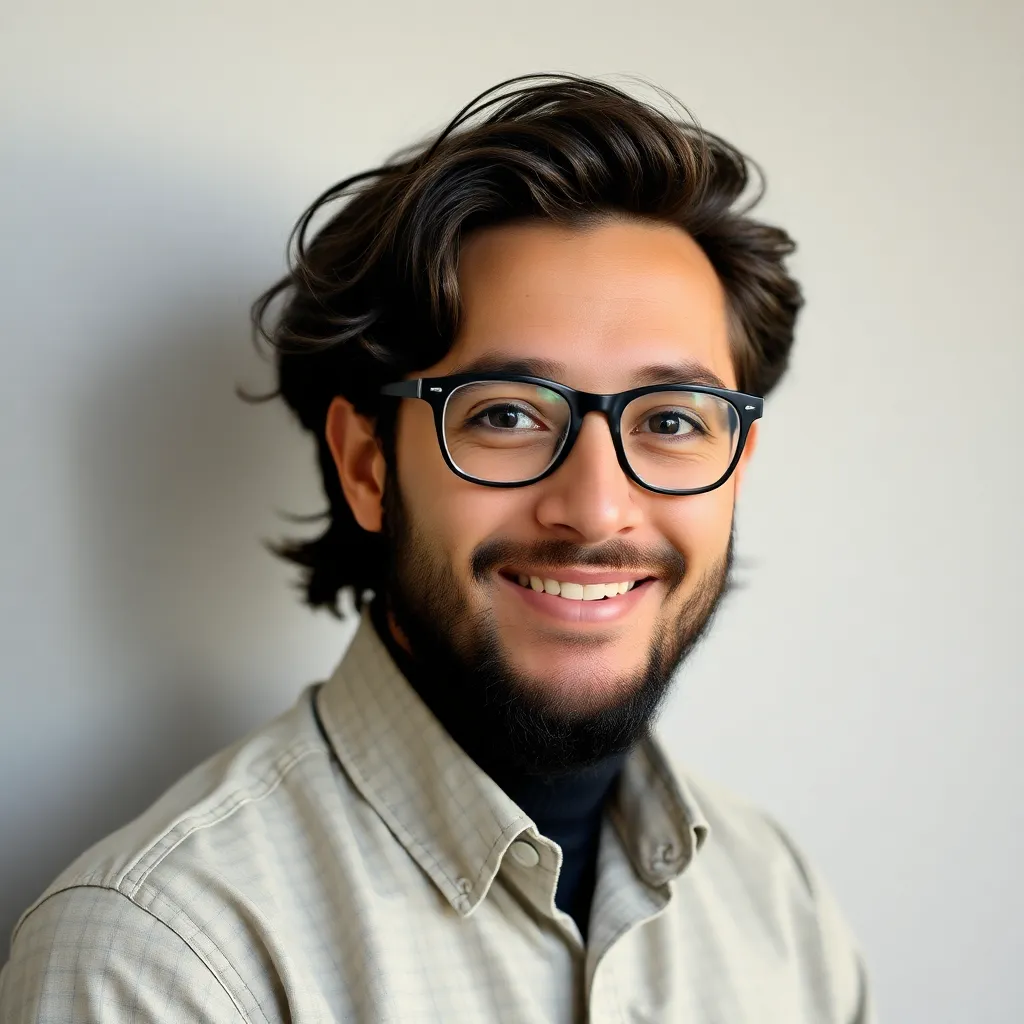
Holbox
May 08, 2025 · 6 min read
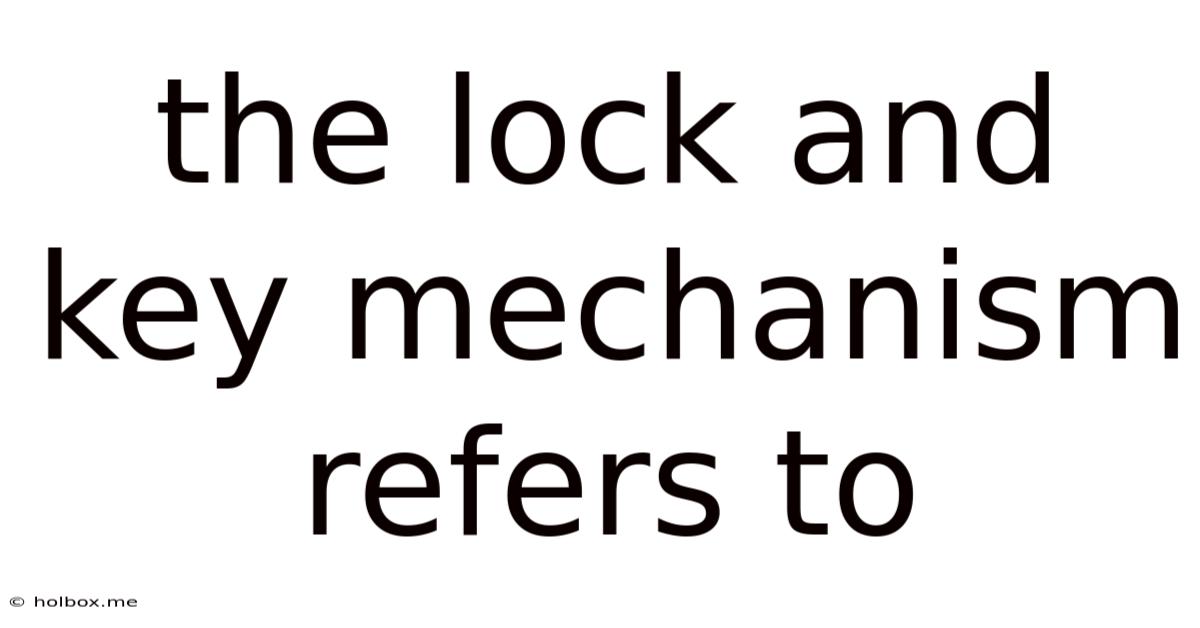
Table of Contents
- The Lock And Key Mechanism Refers To
- Table of Contents
- The Lock and Key Mechanism: A Deep Dive into Molecular Interactions
- The Genesis of the Lock and Key Model
- The Core Analogy Explained
- The Importance of Shape Complementarity
- Limitations of the Lock and Key Model
- The Induced Fit Model: A Refinement of the Lock and Key Concept
- Induced Fit Explained
- Advantages of the Induced Fit Model
- Applications of the Lock and Key and Induced Fit Models
- 1. Enzyme Catalysis:
- 2. Drug Design:
- 3. Receptor-Ligand Interactions:
- 4. Antibody-Antigen Interactions:
- Expanding the Understanding: Beyond the Simple Models
- Considering Dynamic Interactions:
- The Role of Water Molecules:
- Computational Modeling:
- Conclusion: A Continuing Evolution
- Latest Posts
- Latest Posts
- Related Post
The Lock and Key Mechanism: A Deep Dive into Molecular Interactions
The "lock and key" mechanism is a cornerstone concept in biochemistry, explaining the highly specific interactions between biological molecules, primarily enzymes and their substrates. While a simplified model, it provides a fundamental understanding of how biological processes are regulated with exquisite precision. This article will explore the lock and key mechanism in detail, examining its origins, limitations, and its relevance across various biological contexts. We'll also delve into the more nuanced and accurate "induced fit" model, which builds upon the original lock and key concept.
The Genesis of the Lock and Key Model
The lock and key analogy was first proposed by Emil Fischer in 1894, while studying the specificity of enzyme-substrate interactions. Fischer, a renowned chemist, observed that enzymes exhibited remarkable selectivity, catalyzing reactions with only specific molecules while ignoring others. This highly specific interaction reminded him of a lock and its corresponding key: just as a specific key fits only into its corresponding lock, a specific enzyme only binds to its complementary substrate.
The Core Analogy Explained
The lock represents the enzyme, a biological catalyst with a precisely defined three-dimensional structure, featuring an active site. This active site is a pocket or cleft on the enzyme's surface, possessing a unique shape and chemical properties. The key represents the substrate, the molecule upon which the enzyme acts. The substrate's shape and chemical properties must precisely complement those of the enzyme's active site for binding to occur. Only when the substrate fits perfectly into the active site does the enzyme-substrate complex form, initiating the catalytic process.
The Importance of Shape Complementarity
The success of the lock and key model lies in emphasizing the crucial role of shape complementarity. The substrate must possess a shape that is geometrically compatible with the active site. Furthermore, the chemical properties of the substrate's functional groups must interact favorably with the amino acid residues lining the active site. These interactions—hydrogen bonds, ionic interactions, van der Waals forces, and hydrophobic interactions—contribute to the high affinity and specificity of enzyme-substrate binding.
Limitations of the Lock and Key Model
Despite its intuitive simplicity and initial success in explaining enzyme specificity, the lock and key model has limitations. It fails to account for several observations regarding enzyme-substrate interactions:
- Enzyme Flexibility: The model assumes both the enzyme and substrate are rigid structures. However, both molecules possess a degree of flexibility and can undergo conformational changes.
- Induced Fit: Experimental evidence demonstrates that enzymes often undergo conformational changes upon substrate binding, further optimizing the interaction.
- Transition State Stabilization: The model doesn't explicitly explain how enzymes stabilize the transition state, the high-energy intermediate formed during the reaction. Enzyme catalysis relies heavily on this stabilization, which reduces the activation energy of the reaction.
The Induced Fit Model: A Refinement of the Lock and Key Concept
To address the shortcomings of the lock and key model, Daniel Koshland proposed the induced fit model in 1958. This model acknowledges the flexibility of both enzymes and substrates. It suggests that the enzyme's active site is not a rigid, pre-formed structure but rather a flexible entity that adapts to the substrate upon binding.
Induced Fit Explained
The induced fit model proposes that the initial interaction between enzyme and substrate is relatively weak. This initial binding induces conformational changes in both the enzyme and substrate, leading to a more complementary and tighter fit. This conformational change optimizes the positioning of catalytic residues within the active site, maximizing the efficiency of the catalytic process.
Advantages of the Induced Fit Model
The induced fit model offers several advantages over the lock and key model:
- Explains Enzyme Flexibility: It accommodates the inherent flexibility of both the enzyme and substrate.
- Accounts for Conformational Changes: It explicitly describes the conformational changes that occur upon substrate binding, leading to tighter binding and enhanced catalysis.
- Provides a Mechanism for Transition State Stabilization: The induced fit allows the enzyme to adopt a conformation that stabilizes the transition state, thus lowering the activation energy.
- Explains Substrate Specificity: The conformational changes contribute to the high substrate specificity of enzymes.
Applications of the Lock and Key and Induced Fit Models
The concepts of lock and key and induced fit are not merely theoretical constructs; they have far-reaching implications in various biological contexts:
1. Enzyme Catalysis:
The core application lies in understanding how enzymes catalyze biological reactions with remarkable speed and selectivity. The models explain the mechanism behind enzyme-substrate specificity and the efficiency of catalysis.
2. Drug Design:
The principles of molecular recognition, central to both models, form the foundation of rational drug design. By designing drugs that mimic the shape and charge of substrates or inhibitors, pharmaceutical scientists can create medications that bind specifically to target molecules and elicit desired therapeutic effects. For example, many drugs are designed to fit into the active site of specific enzymes to inhibit their activity.
3. Receptor-Ligand Interactions:
Beyond enzymes, the lock and key and induced fit models apply to other biological interactions, such as those between receptors and ligands. Hormones, neurotransmitters, and other signaling molecules bind to specific receptors to trigger cellular responses. These interactions also involve shape complementarity and often conformational changes, reflecting the principles of the induced fit model.
4. Antibody-Antigen Interactions:
The immune system relies on highly specific interactions between antibodies and antigens. Antibodies recognize and bind to specific foreign molecules (antigens) with exceptional precision. This binding often involves induced fit, where the antibody's structure adapts to the antigen, enhancing the strength and specificity of the interaction.
Expanding the Understanding: Beyond the Simple Models
While the lock and key and induced fit models provide invaluable frameworks for understanding molecular interactions, they are simplified representations of a complex reality. Recent advances in structural biology and computational modeling have revealed that enzyme-substrate interactions are even more dynamic and intricate.
Considering Dynamic Interactions:
The models often overlook the dynamic nature of these interactions. The active site is not a static entity; it's constantly undergoing subtle conformational fluctuations. These fluctuations influence substrate binding, catalytic efficiency, and the overall dynamics of the enzyme-substrate complex.
The Role of Water Molecules:
Water molecules play a crucial, often overlooked, role in enzyme-substrate interactions. They participate in hydrogen bonding, influence the conformational flexibility of both the enzyme and substrate, and sometimes directly participate in the catalytic mechanism itself.
Computational Modeling:
Computational methods, such as molecular dynamics simulations, provide increasingly detailed insights into the dynamic nature of enzyme-substrate interactions. These simulations allow researchers to visualize the conformational changes that occur during binding and catalysis, providing a more comprehensive understanding of these complex processes.
Conclusion: A Continuing Evolution
The lock and key and induced fit models represent landmark achievements in biochemistry, providing fundamental understanding of enzyme-substrate specificity. While these models have limitations, their enduring value lies in their ability to provide a conceptual framework for comprehending the remarkable precision of biological interactions. As our understanding of molecular interactions continues to evolve through advanced experimental techniques and computational modeling, the core principles of these models will remain essential tools in the study of life's processes. The journey toward a complete understanding of these fundamental interactions is ongoing, but the lock and key and induced fit models serve as invaluable stepping stones on the path.
Latest Posts
Latest Posts
-
5000 Sq Ft To Sq M
May 20, 2025
-
5000 Square Feet To Square Meters
May 20, 2025
-
2000 Minutes Is How Many Hours
May 20, 2025
-
How Long Is 1 000 Seconds
May 20, 2025
-
What Is 81 Kilos In Stones
May 20, 2025
Related Post
Thank you for visiting our website which covers about The Lock And Key Mechanism Refers To . We hope the information provided has been useful to you. Feel free to contact us if you have any questions or need further assistance. See you next time and don't miss to bookmark.