The Carbon Nitrogen Peptide Bond Is Rigid
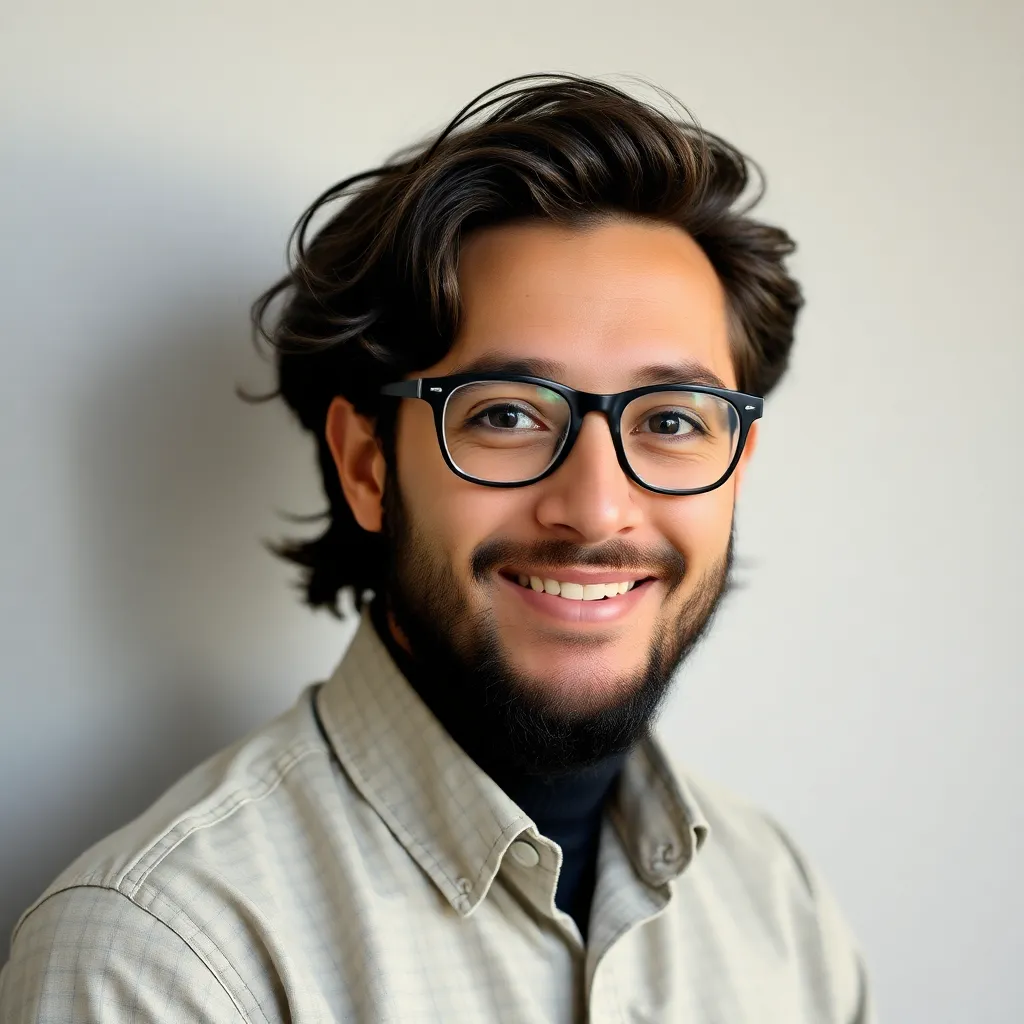
Holbox
Apr 06, 2025 · 6 min read

Table of Contents
- The Carbon Nitrogen Peptide Bond Is Rigid
- Table of Contents
- The Carbon-Nitrogen Peptide Bond: A Rigid Backbone of Life
- Understanding the Peptide Bond: A Chemical Perspective
- Resonance Stabilization and Planarity
- The Trans Configuration: The Dominant Isomer
- Implications of Peptide Bond Rigidity on Protein Structure
- Secondary Structure Formation: Alpha-Helices and Beta-Sheets
- Tertiary and Quaternary Structure: A Consequence of Local Rigidity
- The Role of Peptide Bond Rigidity in Protein Stability
- Exceptions to the Rule: Cis Peptide Bonds and Proline's Unique Role
- Proline's Impact: Breaking the Rules
- Implications of Cis-Proline Isomers
- The Peptide Bond and Protein Engineering: Harnessing Rigidity
- Directed Evolution and Rational Design
- Peptide Bond Mimics: Expanding the Design Space
- Conclusion: The Unsung Hero of Protein Structure
- Latest Posts
- Latest Posts
- Related Post
The Carbon-Nitrogen Peptide Bond: A Rigid Backbone of Life
The peptide bond, the cornerstone of protein structure, is a fundamental element in the intricate machinery of life. Its rigidity, a characteristic often overlooked in broader discussions of protein folding and function, plays a crucial role in dictating the three-dimensional conformation of proteins and, consequently, their biological activities. This article delves into the structural features of the peptide bond, explaining why it exhibits significant rigidity, and exploring the implications of this rigidity on protein structure and function. We will explore the contributing factors to its planarity, the exceptions to this rule, and the impact on protein folding and protein engineering.
Understanding the Peptide Bond: A Chemical Perspective
The peptide bond is an amide linkage formed between the carboxyl group (-COOH) of one amino acid and the amino group (-NH2) of another amino acid. This reaction, a condensation reaction, releases a water molecule, resulting in a covalent bond between the α-carbon of one amino acid and the nitrogen atom of the next. The resulting structure possesses unique chemical properties that contribute to its remarkable rigidity.
Resonance Stabilization and Planarity
The key to understanding the rigidity of the peptide bond lies in its resonance structure. The carbonyl oxygen (C=O) and the amide nitrogen (N-H) participate in resonance, creating a partial double bond character between the carbon and nitrogen atoms. This partial double bond restricts rotation around the C-N bond, resulting in a planar, or nearly planar, configuration. The resonance structure significantly increases the bond order, making the peptide bond stronger and less prone to rotation than a typical single bond. This planarity is a critical determinant of the overall protein structure.
The Trans Configuration: The Dominant Isomer
Two isomeric forms of the peptide bond exist: cis and trans. The trans configuration, where the α-carbon atoms of adjacent amino acids are on opposite sides of the peptide bond, is significantly more stable than the cis configuration. This is primarily due to steric hindrance in the cis isomer, where the side chains of adjacent amino acids clash. The trans configuration, with its reduced steric clash, is overwhelmingly favored, accounting for more than 99% of peptide bonds in naturally occurring proteins. This preference further contributes to the predictable and relatively rigid nature of the peptide backbone.
Implications of Peptide Bond Rigidity on Protein Structure
The rigidity of the peptide bond profoundly impacts protein structure at various levels. Its planar nature dictates the local conformation of the polypeptide chain, influencing the formation of secondary structures like α-helices and β-sheets.
Secondary Structure Formation: Alpha-Helices and Beta-Sheets
The planar peptide bond is crucial in the formation of secondary structures. The consistent geometry of the peptide bond allows for the predictable hydrogen bonding patterns that stabilize α-helices and β-sheets. In an α-helix, the hydrogen bond between the carbonyl oxygen of one amino acid and the amide hydrogen of an amino acid four residues down the chain relies on the planarity of the peptide bond to maintain the helical structure. Similarly, the extended conformation of β-sheets, stabilized by hydrogen bonds between adjacent strands, is dependent on the planarity of the peptide bonds. Without this rigidity, these highly ordered secondary structures would be significantly destabilized.
Tertiary and Quaternary Structure: A Consequence of Local Rigidity
The local rigidity imposed by the peptide bond influences the higher-order structures of proteins. The constraints on the backbone dihedral angles (φ and ψ angles) resulting from the planarity of the peptide bond significantly reduce the conformational freedom of the polypeptide chain. This limitation, however, is not entirely restrictive; it merely guides the folding pathway, allowing for a more predictable and often more stable three-dimensional structure. The specific arrangement of amino acids along the chain and their interactions with each other (hydrophobic interactions, hydrogen bonds, disulfide bonds, etc.) dictate the final three-dimensional structure. This 3D arrangement, in turn, determines the protein's function.
The Role of Peptide Bond Rigidity in Protein Stability
The rigid nature of the peptide bond contributes significantly to the overall stability of the protein. This rigidity limits conformational flexibility, preventing the protein from easily adopting unfolded or misfolded states. This stability is crucial for maintaining the protein's biological activity and preventing aggregation or degradation. The tightly packed nature of the folded protein structure further strengthens the overall rigidity, making the protein resistant to external denaturing agents.
Exceptions to the Rule: Cis Peptide Bonds and Proline's Unique Role
While the trans configuration is overwhelmingly prevalent, some exceptions do exist. Cis peptide bonds are less common but can occur, particularly near proline residues.
Proline's Impact: Breaking the Rules
Proline, a unique amino acid with its cyclic structure, introduces significant conformational constraints to the polypeptide chain. The cyclic structure of proline restricts the rotation around the N-Cα bond, impacting the planarity of the peptide bond. Proline can adopt both cis and trans conformations with relatively similar energy levels, leading to a higher probability of observing cis-proline peptide bonds compared to other amino acids. The presence of cis-proline peptide bonds can have significant implications on protein folding and function, often introducing kinks or bends in the polypeptide chain.
Implications of Cis-Proline Isomers
The presence of cis-proline peptide bonds can be crucial for protein function. In some cases, a cis-proline isomer is essential for proper protein folding or the formation of specific active sites. The isomerization between cis and trans forms of proline peptide bonds can also play a role in regulating protein activity. The slower isomerization kinetics can introduce a rate-limiting step in some protein folding processes or serve as a switch regulating protein function.
The Peptide Bond and Protein Engineering: Harnessing Rigidity
The understanding of peptide bond rigidity has profound implications in protein engineering. Scientists can leverage this knowledge to design proteins with specific structural properties or enhanced stability.
Directed Evolution and Rational Design
Modern protein engineering techniques, like directed evolution and rational design, utilize knowledge of peptide bond rigidity to manipulate protein structure and function. By introducing mutations or modifying amino acid sequences, researchers can subtly alter the conformation of a protein while preserving the overall stability and function, or even creating entirely new proteins with desirable traits.
Peptide Bond Mimics: Expanding the Design Space
Researchers are also exploring the creation of peptide bond mimics – non-peptide linkages that replicate some of the crucial properties of the peptide bond, such as rigidity and planarity. These mimics can improve protein stability, resistance to proteases (enzymes that degrade proteins), and overall bioactivity.
Conclusion: The Unsung Hero of Protein Structure
The rigidity of the carbon-nitrogen peptide bond is a fundamental property with far-reaching consequences for protein structure and function. Its planar nature, largely due to resonance stabilization, directs the folding pathway, facilitates the formation of secondary structures, contributes to protein stability, and even influences protein dynamics. While exceptions to the rule, such as cis-proline isomers, exist, they only underscore the remarkable role of peptide bond rigidity in shaping the diverse world of proteins. The continuing investigation of this critical bond continues to inform the fields of structural biology, biochemistry, and protein engineering, driving innovation in drug discovery, materials science, and biotechnology. Further research into the nuances of peptide bond behavior is essential for advancing our understanding of biological systems and engineering new, more stable and functional proteins.
Latest Posts
Latest Posts
-
What Happens To The Brightness Of Bulb A
Apr 10, 2025
-
Neo Freudians Place Greater Emphasis On The Blank Than Did Freud
Apr 10, 2025
-
Clot Retraction Is Reported With Code
Apr 10, 2025
-
Statement Of Cash Flows For Sioux A Limited Company
Apr 10, 2025
-
Splinter Results In Tissue Damage Bacteria Enter Wound And Blood
Apr 10, 2025
Related Post
Thank you for visiting our website which covers about The Carbon Nitrogen Peptide Bond Is Rigid . We hope the information provided has been useful to you. Feel free to contact us if you have any questions or need further assistance. See you next time and don't miss to bookmark.