The Bonding Properties Of An Atom Are Determined By Its
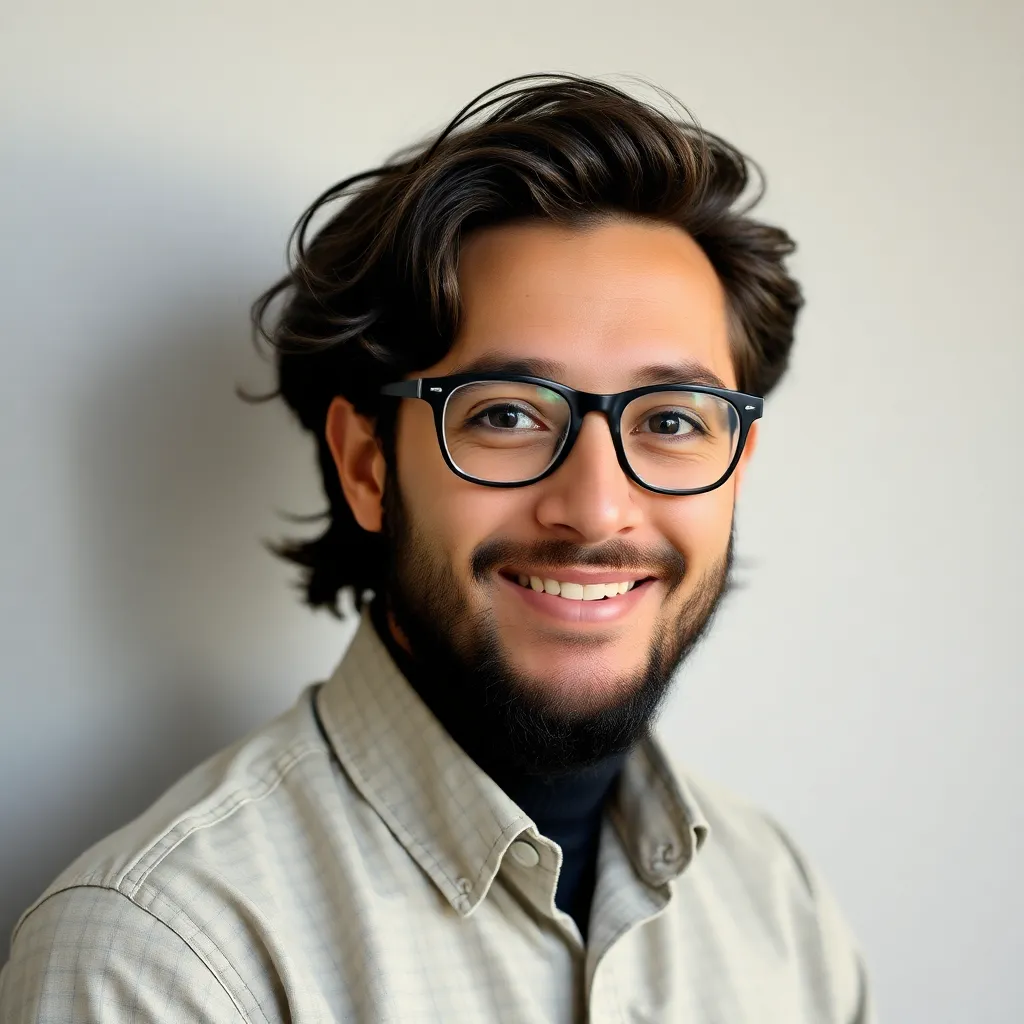
Holbox
May 11, 2025 · 7 min read

Table of Contents
- The Bonding Properties Of An Atom Are Determined By Its
- Table of Contents
- The Bonding Properties of an Atom are Determined by its Electron Configuration
- Understanding Electron Configuration
- Valence Electrons and their Role in Bonding
- Types of Chemical Bonds and their Relationship to Electron Configuration
- 1. Ionic Bonds: Transfer of Electrons
- 2. Covalent Bonds: Sharing of Electrons
- 3. Metallic Bonds: Delocalized Electrons
- 4. Hydrogen Bonds: Special Case of Dipole-Dipole Interaction
- Influence of Electron Configuration on Bonding Properties: Examples
- Conclusion: Electron Configuration as a Cornerstone of Chemical Bonding
- Latest Posts
- Related Post
The Bonding Properties of an Atom are Determined by its Electron Configuration
The behavior of atoms, particularly their tendency to form chemical bonds with other atoms, is fundamentally governed by their electron configuration. This configuration, the arrangement of electrons in the various energy levels and sublevels within an atom, dictates an atom's valence electrons, which are the outermost electrons and are directly involved in chemical bonding. Understanding the electron configuration is, therefore, crucial to comprehending the properties of chemical bonds, which in turn underpins our understanding of the entire material world.
Understanding Electron Configuration
Before diving into the intricacies of bonding, let's establish a firm grasp on electron configuration. Electrons occupy specific energy levels (also known as shells or principal quantum numbers, designated by n = 1, 2, 3, and so on), each capable of holding a limited number of electrons. Within each energy level, there are sublevels (designated by s, p, d, and f), each with its own specific shape and capacity for electrons.
- The s sublevel: Can hold a maximum of 2 electrons.
- The p sublevel: Can hold a maximum of 6 electrons.
- The d sublevel: Can hold a maximum of 10 electrons.
- The f sublevel: Can hold a maximum of 14 electrons.
The filling of these sublevels follows specific rules:
- Aufbau Principle: Electrons fill the lowest energy levels first. This means that 1s fills before 2s, 2s before 2p, and so on.
- Pauli Exclusion Principle: Each orbital (a region within a sublevel where an electron is most likely to be found) can hold a maximum of two electrons, and these electrons must have opposite spins.
- Hund's Rule: Within a subshell, electrons will individually occupy each orbital before doubling up in any one orbital. This minimizes electron-electron repulsion.
By applying these rules, we can determine the electron configuration of any atom. For example, the electron configuration of oxygen (atomic number 8) is 1s²2s²2p⁴. This indicates that oxygen has two electrons in the 1s sublevel, two in the 2s sublevel, and four in the 2p sublevel.
Valence Electrons and their Role in Bonding
The valence electrons, the electrons in the outermost energy level, are the key players in chemical bonding. These electrons are loosely held by the nucleus and are readily available to participate in interactions with other atoms. The number of valence electrons determines an atom's reactivity and the type of bonds it can form.
Atoms strive to achieve a stable electron configuration, often resembling that of the nearest noble gas (Group 18 elements). Noble gases have completely filled outermost electron shells, making them exceptionally unreactive. This tendency to achieve a stable electron configuration is the driving force behind chemical bonding.
Types of Chemical Bonds and their Relationship to Electron Configuration
Several types of chemical bonds exist, each arising from different interactions between valence electrons:
1. Ionic Bonds: Transfer of Electrons
Ionic bonds are formed through the complete transfer of one or more electrons from one atom to another. This transfer creates ions: positively charged cations (atoms that have lost electrons) and negatively charged anions (atoms that have gained electrons). The electrostatic attraction between these oppositely charged ions forms the ionic bond.
Ionic bonds typically occur between atoms with significantly different electronegativities. Electronegativity is a measure of an atom's ability to attract electrons in a chemical bond. Atoms with low electronegativity (like alkali metals) readily lose electrons, while atoms with high electronegativity (like halogens) readily gain electrons. For example, the reaction between sodium (Na) and chlorine (Cl) results in the formation of sodium chloride (NaCl), where Na loses one electron to become Na⁺ and Cl gains one electron to become Cl⁻. The electrostatic attraction between Na⁺ and Cl⁻ forms the ionic bond.
2. Covalent Bonds: Sharing of Electrons
Covalent bonds are formed through the sharing of one or more pairs of electrons between atoms. This sharing allows both atoms to achieve a more stable electron configuration. Covalent bonds typically occur between atoms with similar electronegativities, often nonmetals.
The shared electrons are attracted to the nuclei of both atoms, holding them together. The strength of a covalent bond depends on the number of shared electron pairs and the electronegativity difference between the atoms. A single bond involves one shared electron pair, a double bond involves two shared pairs, and a triple bond involves three shared pairs. For example, in a water molecule (H₂O), each hydrogen atom shares one electron pair with the oxygen atom, resulting in two covalent bonds.
3. Metallic Bonds: Delocalized Electrons
Metallic bonds are found in metals and are characterized by the delocalization of valence electrons. In a metallic solid, the valence electrons are not associated with any particular atom but rather move freely throughout the entire metal lattice. This "sea" of delocalized electrons accounts for the characteristic properties of metals, such as their excellent electrical and thermal conductivity, malleability, and ductility.
The strong electrostatic attraction between the positively charged metal ions and the delocalized electrons holds the metal lattice together. The strength of the metallic bond depends on the number of valence electrons and the size of the metal ions.
4. Hydrogen Bonds: Special Case of Dipole-Dipole Interaction
Hydrogen bonds are a special type of dipole-dipole interaction that occurs between molecules containing a hydrogen atom bonded to a highly electronegative atom (such as oxygen, nitrogen, or fluorine). The highly electronegative atom pulls the shared electrons away from the hydrogen atom, creating a partial positive charge (δ+) on the hydrogen and a partial negative charge (δ-) on the electronegative atom. This creates a dipole moment. The positive end of one molecule is then attracted to the negative end of another molecule, forming a hydrogen bond. Hydrogen bonds are weaker than ionic or covalent bonds but play crucial roles in many biological systems, influencing the properties of water and the structure of proteins and DNA.
Influence of Electron Configuration on Bonding Properties: Examples
Let's examine specific examples to illustrate the strong connection between electron configuration and bonding properties:
-
Sodium (Na): With an electron configuration of [Ne]3s¹, sodium has one valence electron. It readily loses this electron to achieve a stable noble gas configuration ([Ne]), forming a +1 cation (Na⁺) and readily participating in ionic bonding.
-
Chlorine (Cl): With an electron configuration of [Ne]3s²3p⁵, chlorine has seven valence electrons. It readily gains one electron to achieve a stable noble gas configuration ([Ar]), forming a -1 anion (Cl⁻) and readily participating in ionic bonding.
-
Carbon (C): With an electron configuration of [He]2s²2p², carbon has four valence electrons. It readily forms covalent bonds by sharing its valence electrons with other atoms to achieve a stable octet (eight electrons in its outermost shell). This explains carbon's ability to form long chains and complex molecules, the foundation of organic chemistry.
-
Oxygen (O): With an electron configuration of [He]2s²2p⁴, oxygen has six valence electrons. It commonly forms two covalent bonds to achieve a stable octet. This explains the formation of water (H₂O), where oxygen forms two covalent bonds with hydrogen atoms.
Conclusion: Electron Configuration as a Cornerstone of Chemical Bonding
The electron configuration of an atom serves as a fundamental determinant of its bonding properties. By understanding the arrangement of electrons, particularly the valence electrons, we can predict the type of bonds an atom is likely to form and its overall reactivity. This knowledge is essential for understanding and predicting the properties of molecules, materials, and the vast array of chemical reactions that shape our world. The interplay between electron configuration and bonding is a cornerstone of chemistry, driving the formation of diverse compounds and influencing their properties in ways that are both fascinating and essential to our understanding of the universe. From the simplest ionic compounds to the most complex biological molecules, the principles outlined here remain fundamental and provide a powerful framework for explaining and predicting chemical behavior.
Latest Posts
Related Post
Thank you for visiting our website which covers about The Bonding Properties Of An Atom Are Determined By Its . We hope the information provided has been useful to you. Feel free to contact us if you have any questions or need further assistance. See you next time and don't miss to bookmark.