The Active Site Of An Enzyme Is The Region That
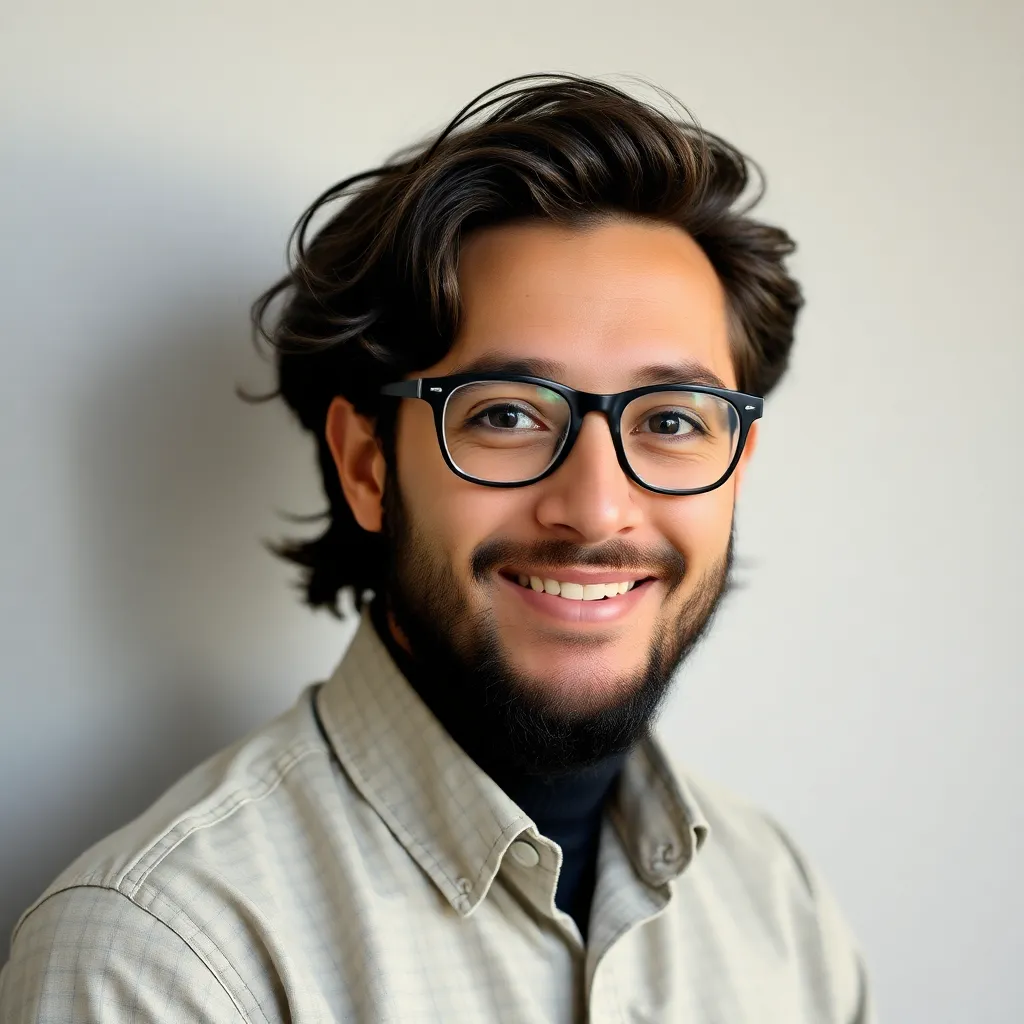
Holbox
Apr 14, 2025 · 7 min read

Table of Contents
- The Active Site Of An Enzyme Is The Region That
- Table of Contents
- The Active Site of an Enzyme: The Region That Makes Life Possible
- The Architecture of the Active Site: A Three-Dimensional Puzzle
- 1. Specific Amino Acid Residues: The Key Players
- 2. Binding Pocket: Holding the Substrate in Place
- 3. Catalytic Residues: The Reaction Initiators
- Enzyme Specificity: The Active Site's Role
- Lock and Key Model: A Simple Analogy
- Induced Fit Model: A More Realistic Perspective
- Factors Affecting Active Site Function
- 1. Temperature: Finding the Goldilocks Zone
- 2. pH: Maintaining the Right Balance
- 3. Inhibitors: Blocking the Active Site
- The Active Site in Action: Examples Across Biology
- 1. Digestive Enzymes: Breaking Down Food
- 2. Metabolic Enzymes: Powering Cellular Processes
- 3. DNA Polymerases: Replicating Genetic Material
- 4. Drug Target Enzymes: A Focus for Pharmaceutical Research
- Studying the Active Site: Techniques and Approaches
- 1. X-ray Crystallography: Visualizing the Structure
- 2. Nuclear Magnetic Resonance (NMR) Spectroscopy: Studying Dynamics
- 3. Site-Directed Mutagenesis: Modifying the Active Site
- 4. Computational Modeling: Predicting and Simulating Interactions
- Conclusion: The Active Site – A Microcosm of Life's Processes
- Latest Posts
- Latest Posts
- Related Post
The Active Site of an Enzyme: The Region That Makes Life Possible
The active site of an enzyme is the region that catalyzes biological reactions. It's a crucial area within the enzyme's three-dimensional structure, responsible for binding substrate molecules and facilitating the chemical transformations necessary for life. Understanding the active site's properties and function is paramount to comprehending the intricate mechanisms of biological processes, from digestion to DNA replication. This article delves deep into the intricacies of the enzyme active site, exploring its characteristics, functionality, and significance in various biological contexts.
The Architecture of the Active Site: A Three-Dimensional Puzzle
The active site isn't simply a random cluster of amino acids. Instead, it's a precisely sculpted three-dimensional region, often a cleft or pocket within the enzyme's larger structure. This precise architecture is essential for its function. Several key features define the active site:
1. Specific Amino Acid Residues: The Key Players
The active site comprises a specific arrangement of amino acid side chains, often from different parts of the polypeptide chain. These residues are not randomly positioned; their spatial arrangement is crucial for substrate binding and catalysis. Some residues directly participate in the catalytic mechanism, while others contribute to substrate binding and orientation. The unique combination of these residues determines the enzyme's specificity and catalytic efficiency. Think of it as a lock and key mechanism, where the substrate is the key and the active site is the lock. Only the right key (substrate) can fit perfectly into the lock (active site) to initiate the reaction.
2. Binding Pocket: Holding the Substrate in Place
The active site often forms a pocket or cleft that precisely accommodates the substrate molecule. This binding pocket uses various non-covalent interactions, including hydrogen bonds, van der Waals forces, hydrophobic interactions, and sometimes ionic interactions, to hold the substrate in place. The strength and specificity of these interactions determine the enzyme's affinity for its substrate. The tighter the fit, the more efficiently the enzyme can catalyze the reaction.
3. Catalytic Residues: The Reaction Initiators
Within the active site, specific amino acid residues act as catalytic residues. These residues directly participate in the chemical transformation of the substrate. They can perform a variety of functions, including:
- Acid-base catalysis: Donating or accepting protons to facilitate the reaction.
- Covalent catalysis: Forming a temporary covalent bond with the substrate, creating a reactive intermediate.
- Metal ion catalysis: Utilizing metal ions to stabilize transition states or participate in redox reactions.
The precise mechanism of catalysis varies widely depending on the enzyme and the reaction it catalyzes.
Enzyme Specificity: The Active Site's Role
The active site's unique three-dimensional structure is directly responsible for an enzyme's specificity. Enzymes generally exhibit remarkable selectivity, catalyzing only a specific reaction or a narrow range of reactions. This specificity is a result of the precise interactions between the substrate and the active site. This is often described using the "lock and key" model and the more refined "induced fit" model.
Lock and Key Model: A Simple Analogy
This classic model depicts the substrate as a key that fits precisely into the active site's lock. Only the correct substrate with the complementary shape and chemical properties can bind effectively. This model explains the high specificity of many enzymes but doesn't fully account for the flexibility observed in some enzyme-substrate interactions.
Induced Fit Model: A More Realistic Perspective
The induced fit model suggests that the enzyme's active site is initially flexible and undergoes a conformational change upon substrate binding. This conformational change optimizes the interactions between the enzyme and the substrate, facilitating catalysis. The substrate doesn't simply fit into a rigid active site; it induces a change that enhances the catalytic efficiency. This model accounts for the enzyme's ability to accommodate slightly different substrates while maintaining a degree of specificity.
Factors Affecting Active Site Function
Several factors can influence the activity of an enzyme's active site:
1. Temperature: Finding the Goldilocks Zone
Enzymes generally have an optimal temperature range for activity. At low temperatures, the reaction rate is slow due to reduced kinetic energy. At high temperatures, the enzyme can denature, losing its three-dimensional structure and consequently its catalytic activity. The active site's conformation is directly affected by temperature, impacting its ability to bind substrate and catalyze the reaction.
2. pH: Maintaining the Right Balance
Similar to temperature, pH also significantly impacts the enzyme's activity. Changes in pH can alter the charge of amino acid side chains in the active site, affecting substrate binding and the catalytic mechanism. Each enzyme has an optimal pH range where its active site is correctly structured and functional.
3. Inhibitors: Blocking the Active Site
Enzyme inhibitors are molecules that can bind to the active site, preventing substrate binding or interfering with the catalytic mechanism. Inhibitors can be competitive (competing with the substrate for the active site) or non-competitive (binding to a different site, altering the active site's conformation). Inhibitors play crucial roles in regulating enzyme activity and are important targets for drug development.
The Active Site in Action: Examples Across Biology
The active site’s role extends across diverse biological processes. Let's examine some key examples:
1. Digestive Enzymes: Breaking Down Food
Digestive enzymes, such as amylase (breaks down starch), protease (breaks down proteins), and lipase (breaks down fats), each have highly specialized active sites tailored to their respective substrates. These active sites facilitate the hydrolysis reactions necessary for nutrient absorption.
2. Metabolic Enzymes: Powering Cellular Processes
Metabolic enzymes play vital roles in cellular metabolism, catalyzing the reactions involved in energy production, biosynthesis, and waste removal. Examples include enzymes involved in glycolysis, the citric acid cycle, and oxidative phosphorylation. Their active sites are finely tuned to facilitate the intricate chemical transformations required for cellular function.
3. DNA Polymerases: Replicating Genetic Material
DNA polymerases are enzymes responsible for replicating DNA. Their active sites are meticulously designed to bind to DNA nucleotides, ensuring accurate replication of the genetic code. The high fidelity of DNA replication depends on the precise interaction between the active site and the nucleotides.
4. Drug Target Enzymes: A Focus for Pharmaceutical Research
Many drugs target specific enzymes by interacting with their active sites. By inhibiting or activating these enzymes, drugs can effectively treat various diseases. For example, many antiviral drugs target viral enzymes essential for viral replication.
Studying the Active Site: Techniques and Approaches
Investigating the structure and function of enzyme active sites requires a multidisciplinary approach involving various techniques:
1. X-ray Crystallography: Visualizing the Structure
X-ray crystallography is a powerful technique for determining the three-dimensional structure of proteins, including the enzyme's active site. By analyzing the diffraction pattern of X-rays scattered by enzyme crystals, researchers can build a detailed model of the active site's structure and identify the amino acid residues involved.
2. Nuclear Magnetic Resonance (NMR) Spectroscopy: Studying Dynamics
NMR spectroscopy provides information on the dynamics and flexibility of the active site. It can reveal how the active site's structure changes upon substrate binding and during catalysis.
3. Site-Directed Mutagenesis: Modifying the Active Site
Site-directed mutagenesis involves altering specific amino acids within the active site to study their role in substrate binding and catalysis. By changing individual residues, researchers can assess their contribution to the enzyme's activity and specificity.
4. Computational Modeling: Predicting and Simulating Interactions
Computational techniques, including molecular dynamics simulations, are increasingly used to study enzyme active sites. These simulations can provide insights into the dynamics of substrate binding, the catalytic mechanism, and the effects of mutations or inhibitors.
Conclusion: The Active Site – A Microcosm of Life's Processes
The active site of an enzyme is a remarkable example of nature's precision engineering. Its unique architecture, determined by a specific arrangement of amino acid residues, dictates the enzyme's specificity and catalytic power. Understanding the active site is crucial for comprehending the intricate mechanisms of biological processes and developing new therapeutic strategies. Further research into these fascinating molecular machines promises to reveal more about their remarkable functionality and their vital role in maintaining life itself. The active site isn't just a region within an enzyme; it's the powerhouse driving countless biological reactions and ensuring the seamless functioning of life as we know it.
Latest Posts
Latest Posts
-
Prepare An Income Statement Through Gross Profit
Apr 22, 2025
-
Sequence Is True For The Lytic Cycle Of A Virus
Apr 22, 2025
-
Fill The Series In Range B4 F4 With A Growth Trend
Apr 22, 2025
-
One Important Objective Of Purchasing Is To
Apr 22, 2025
-
A Small Stock Dividend Is Defined As
Apr 22, 2025
Related Post
Thank you for visiting our website which covers about The Active Site Of An Enzyme Is The Region That . We hope the information provided has been useful to you. Feel free to contact us if you have any questions or need further assistance. See you next time and don't miss to bookmark.