Steam Enters A Well Insulated Turbine
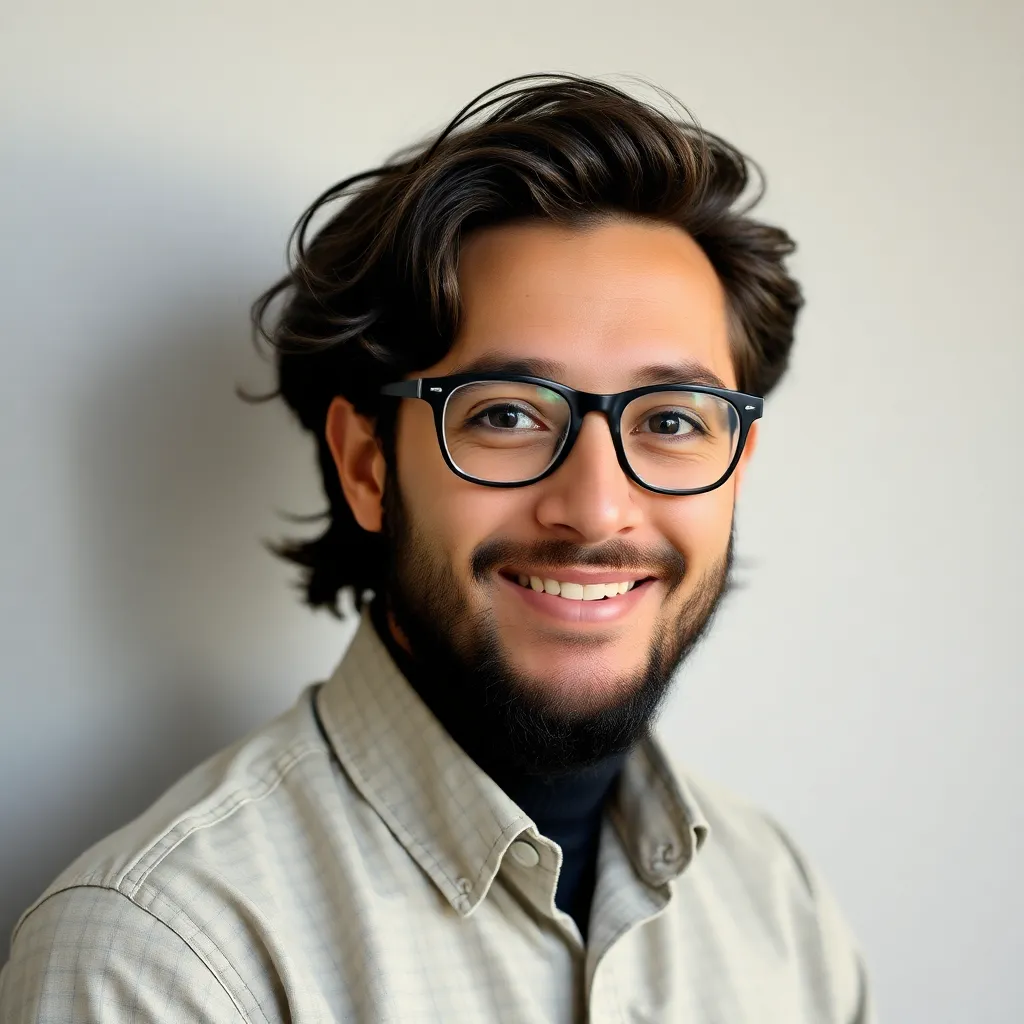
Holbox
Apr 03, 2025 · 6 min read
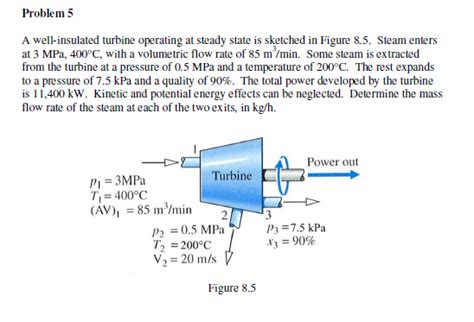
Table of Contents
- Steam Enters A Well Insulated Turbine
- Table of Contents
- Steam Enters a Well-Insulated Turbine: A Deep Dive into Thermodynamics and Efficiency
- Thermodynamic Principles Governing Steam Turbine Operation
- 1. Adiabatic Expansion:
- 2. Isentropic Expansion (Ideal Case):
- 3. Polytropic Expansion (Real-World Scenario):
- 4. Relationship Between Pressure, Volume, and Temperature:
- Energy Transformations Within the Turbine
- 1. Nozzle Expansion:
- 2. Blade Interaction:
- 3. Stage-wise Expansion:
- 4. Exhaust Steam:
- Factors Affecting Turbine Efficiency
- 1. Isentropic Efficiency:
- 2. Blade Design and Manufacturing:
- 3. Steam Quality:
- 4. Leakage:
- 5. Operating Conditions:
- Analyzing Turbine Performance: Calculations and Equations
- Practical Considerations and Applications
- Conclusion
- Latest Posts
- Latest Posts
- Related Post
Steam Enters a Well-Insulated Turbine: A Deep Dive into Thermodynamics and Efficiency
Steam turbines, marvels of engineering, convert the thermal energy of high-pressure steam into mechanical work, driving everything from power plants to industrial machinery. Understanding their operation, particularly within the context of well-insulated systems, is crucial for optimizing efficiency and performance. This article delves into the intricacies of steam flowing through a well-insulated turbine, exploring the thermodynamic principles, energy transformations, and practical considerations involved.
Thermodynamic Principles Governing Steam Turbine Operation
At the heart of a steam turbine's operation lies the fundamental principle of thermodynamics: the conversion of heat energy into mechanical work. When high-pressure, high-temperature steam enters a well-insulated turbine, several key thermodynamic processes unfold:
1. Adiabatic Expansion:
A well-insulated turbine minimizes heat exchange with the surroundings. This means the expansion process is essentially adiabatic – no heat is added or removed during the process. This is a crucial simplification in the analysis, allowing us to utilize simplified thermodynamic relations. The adiabatic assumption simplifies calculations considerably, but in reality, some heat loss is inevitable. However, minimizing heat loss is paramount for maximizing efficiency.
2. Isentropic Expansion (Ideal Case):
In an ideal, isentropic expansion, the entropy of the steam remains constant throughout the process. This is a theoretical idealization. Friction and other irreversibilities always cause some increase in entropy in a real turbine. However, the isentropic efficiency serves as a benchmark for comparing real turbines to their ideal counterparts.
3. Polytropic Expansion (Real-World Scenario):
The actual expansion process within a real turbine is often modeled as polytropic. This accounts for the irreversible losses due to friction, turbulence, and heat transfer (though minimized in a well-insulated design). A polytropic process exhibits a change in entropy, reflecting these real-world imperfections.
4. Relationship Between Pressure, Volume, and Temperature:
The changes in pressure, volume, and temperature of the steam as it expands through the turbine are governed by the steam tables and thermodynamic relationships. Understanding these changes is vital for predicting the turbine's power output and efficiency. The decrease in pressure and temperature is directly related to the work extracted from the steam.
Energy Transformations Within the Turbine
The energy transformations within a well-insulated turbine are central to its functionality. The steam's initial energy, primarily in the form of enthalpy, is converted into kinetic energy as it accelerates through the turbine's stages. This kinetic energy then interacts with the turbine blades, causing them to rotate, ultimately producing mechanical work. The process can be broken down into these key stages:
1. Nozzle Expansion:
Before entering the turbine blades, the high-pressure steam often passes through nozzles. These nozzles accelerate the steam, converting some of its enthalpy into kinetic energy. The design of the nozzles is critical in optimizing the steam velocity and directing its flow onto the blades.
2. Blade Interaction:
The accelerated steam then impacts the turbine blades, transferring its kinetic energy to the rotating shaft. The shape and design of the blades are optimized to extract maximum energy from the steam flow. This involves careful consideration of blade angles and profiles to maximize impulse and reaction forces.
3. Stage-wise Expansion:
Modern turbines employ multiple stages of blades, each extracting a portion of the steam's energy. This stage-wise expansion allows for a more controlled and efficient energy extraction process, reducing stress on individual blades and improving overall efficiency.
4. Exhaust Steam:
After passing through all stages, the steam exits the turbine at a significantly lower pressure and temperature. This exhaust steam still retains some energy, though considerably less than at the inlet. This energy can often be recovered in further stages (reheating) or used in other processes (e.g., process heating).
Factors Affecting Turbine Efficiency
While a well-insulated turbine minimizes heat loss, several other factors influence its overall efficiency:
1. Isentropic Efficiency:
This parameter compares the actual work output of the turbine to the work that would be produced in an ideal, isentropic expansion. A higher isentropic efficiency indicates less energy loss due to irreversibilities.
2. Blade Design and Manufacturing:
The design and precision of the turbine blades directly impact the energy transfer from the steam to the rotor. Improper blade design or manufacturing tolerances can lead to increased friction and reduced efficiency.
3. Steam Quality:
The quality of the steam (the proportion of vapor to liquid) significantly affects its thermodynamic properties and its ability to transfer energy to the turbine blades. Wet steam (containing liquid water) can lead to erosion and reduced efficiency.
4. Leakage:
Leakage of steam past the blades and seals can reduce the overall efficiency. Minimizing leakage through careful sealing and design is crucial.
5. Operating Conditions:
The turbine's operating pressure, temperature, and flow rate affect its performance and efficiency. Optimizing these parameters for specific applications is essential.
Analyzing Turbine Performance: Calculations and Equations
Analyzing turbine performance requires applying thermodynamic principles and equations. While detailed calculations can be complex, some key equations are fundamental:
-
First Law of Thermodynamics (Energy Balance): This fundamental law governs the energy balance in the turbine, relating the enthalpy change of the steam to the work produced and any heat losses (which are minimal in a well-insulated case).
-
Isentropic Relations: These relations are crucial for calculating the theoretical work output under ideal conditions. They relate pressure, temperature, and enthalpy changes during an isentropic process.
-
Polytropic Relations: For real-world scenarios, polytropic relations, which incorporate an efficiency factor, are more realistic in determining actual work output.
-
Specific Volume and Density Calculations: These are essential for calculating mass flow rates and determining the size and design parameters of the turbine.
Practical Considerations and Applications
Steam turbines find extensive applications in various industries:
-
Power Generation: Steam turbines are the backbone of many power plants, converting the thermal energy from fossil fuels, nuclear reactions, or geothermal sources into electricity.
-
Industrial Processes: They are used to drive pumps, compressors, and other industrial equipment, providing mechanical power for various manufacturing processes.
-
Marine Propulsion: Steam turbines power many large ships and naval vessels, offering reliable and efficient propulsion systems.
-
Combined Cycle Power Plants: Steam turbines are often integrated into combined cycle power plants, boosting overall efficiency by utilizing the exhaust heat from gas turbines to generate additional power.
Conclusion
Steam entering a well-insulated turbine represents a complex interplay of thermodynamic principles and engineering design. Understanding the adiabatic expansion, energy transformations, and factors affecting efficiency is crucial for optimizing turbine performance. While the ideal isentropic expansion provides a theoretical benchmark, the reality involves polytropic expansion accounting for inherent irreversibilities. The design of nozzles, blades, and the overall turbine configuration directly impacts energy extraction and efficiency. By carefully considering these factors, engineers can design and operate highly efficient steam turbines for a variety of applications, playing a vital role in power generation and industrial processes worldwide. Continuous advancements in materials science and computational fluid dynamics are leading to further improvements in turbine design and efficiency, driving us towards more sustainable and efficient energy systems.
Latest Posts
Latest Posts
-
Yellow Bone Marrow Contains A Large Percentage Of
Apr 07, 2025
-
Which Protocol Is Used To Create Subscriptions
Apr 07, 2025
-
For A Monopolistically Competitive Firm Marginal Revenue
Apr 07, 2025
-
You Build A Chicken Coop In Your Suburban Backyard
Apr 07, 2025
-
Readings For Diversity And Social Justice
Apr 07, 2025
Related Post
Thank you for visiting our website which covers about Steam Enters A Well Insulated Turbine . We hope the information provided has been useful to you. Feel free to contact us if you have any questions or need further assistance. See you next time and don't miss to bookmark.