Select The Appropriate Reagents To Achieve The Synthetic Transformation Shown
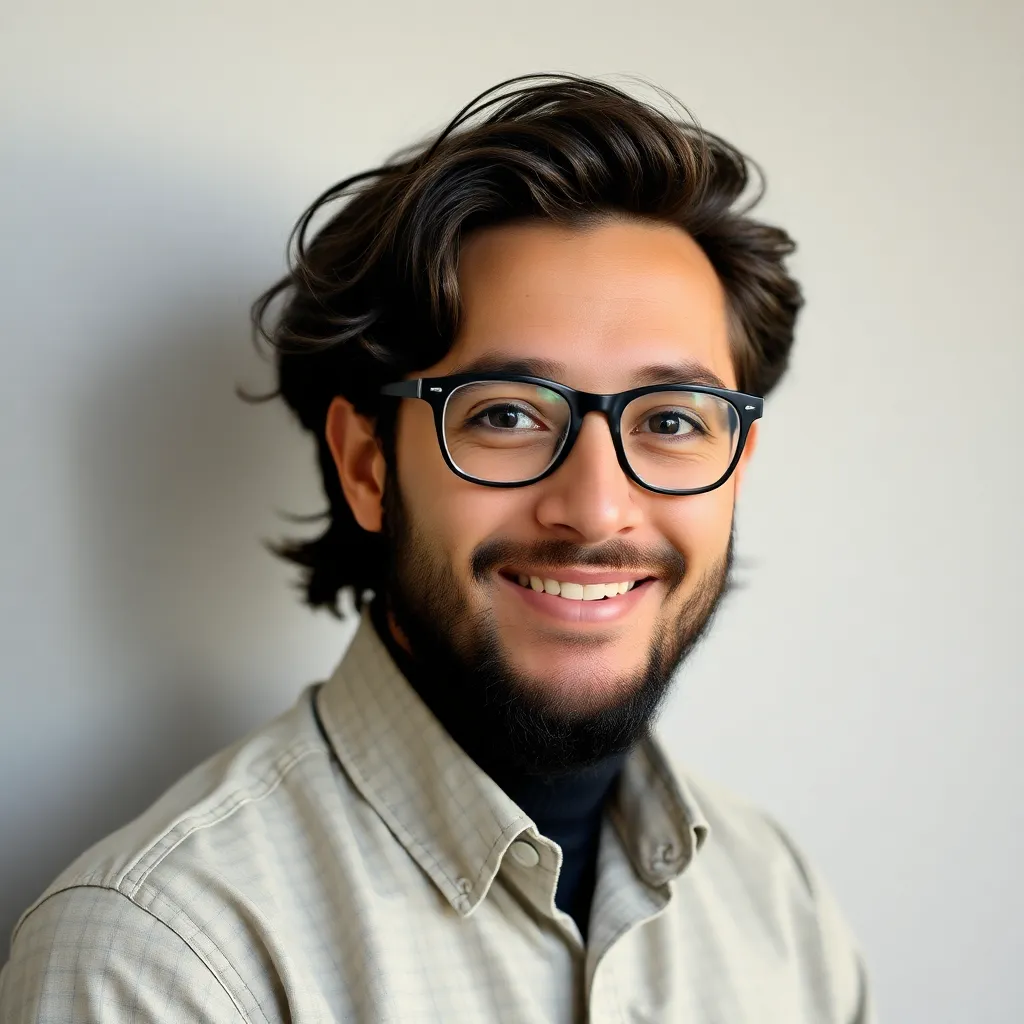
Holbox
May 08, 2025 · 6 min read
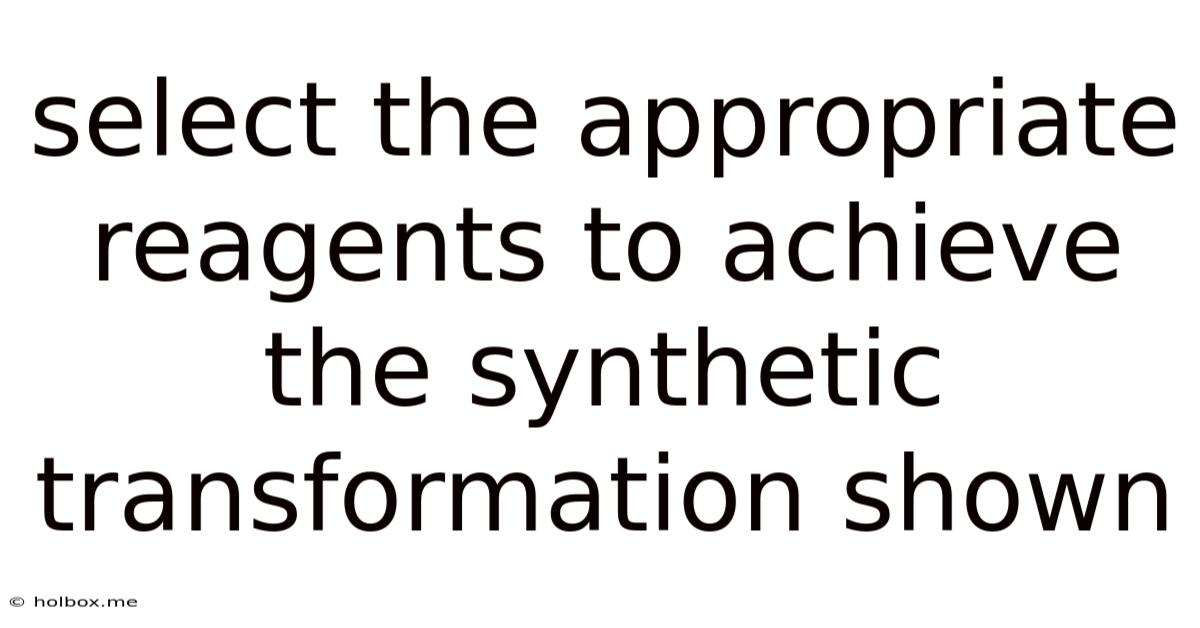
Table of Contents
- Select The Appropriate Reagents To Achieve The Synthetic Transformation Shown
- Table of Contents
- Selecting the Appropriate Reagents for Organic Synthesis: A Comprehensive Guide
- Understanding Reaction Mechanisms: The Foundation of Reagent Selection
- SN1 vs. SN2 Reactions: A Case Study in Reagent Selection
- Electrophilic Aromatic Substitution: Directing Groups and Reagent Choice
- Functional Group Transformations: A Reagent-Centric Approach
- Oxidation and Reduction Reactions
- Protecting Groups: Safeguarding Functional Groups
- Practical Considerations in Reagent Selection: Beyond the Theory
- Case Studies: Applying Reagent Selection Principles
- Conclusion: A Continuous Learning Process
- Latest Posts
- Related Post
Selecting the Appropriate Reagents for Organic Synthesis: A Comprehensive Guide
Organic synthesis, the art and science of constructing complex organic molecules from simpler precursors, hinges on the judicious selection of reagents. Choosing the right reagent is crucial for achieving the desired transformation efficiently, selectively, and with high yield. This process demands a thorough understanding of reaction mechanisms, reagent properties, and potential side reactions. This article delves into the strategies and considerations involved in selecting appropriate reagents for various synthetic transformations, emphasizing practical application and problem-solving.
Understanding Reaction Mechanisms: The Foundation of Reagent Selection
Before diving into specific reagent choices, it's paramount to comprehend the underlying reaction mechanism. The mechanism dictates the pathway by which reactants are converted into products. A deep understanding allows you to anticipate potential challenges and select reagents that facilitate the desired pathway while minimizing unwanted side reactions. For instance, understanding whether a reaction proceeds via SN1, SN2, E1, or E2 mechanisms is crucial in choosing appropriate nucleophiles, electrophiles, and solvents.
SN1 vs. SN2 Reactions: A Case Study in Reagent Selection
Consider the alkyl halide substitution reactions. SN1 reactions (Substitution Nucleophilic Unimolecular) involve a two-step mechanism where the leaving group departs first, forming a carbocation intermediate. This intermediate is then attacked by the nucleophile. SN2 reactions (Substitution Nucleophilic Bimolecular), conversely, are concerted, with the nucleophile attacking the substrate simultaneously as the leaving group departs.
-
SN1 Reactions: Tertiary alkyl halides generally favor SN1 reactions due to the stability of the tertiary carbocation. Weak nucleophiles (e.g., water, alcohols) in protic solvents (e.g., water, methanol) are typically employed to avoid competing SN2 pathways. The choice of solvent is crucial; protic solvents stabilize the carbocation intermediate.
-
SN2 Reactions: Primary alkyl halides are ideal substrates for SN2 reactions. Strong nucleophiles (e.g., hydroxide, cyanide, azide) are needed, and aprotic solvents (e.g., DMSO, DMF) are preferred to minimize solvation of the nucleophile, enhancing its reactivity. Steric hindrance around the electrophilic carbon significantly impacts the reaction rate; highly branched substrates are poor SN2 candidates.
Electrophilic Aromatic Substitution: Directing Groups and Reagent Choice
Electrophilic aromatic substitution is a cornerstone reaction in aromatic chemistry. The nature of the substituent already present on the aromatic ring (the directing group) significantly influences the regioselectivity of the reaction. Electron-donating groups (e.g., -OH, -NH2, -OCH3) are ortho/para directing, while electron-withdrawing groups (e.g., -NO2, -COOH, -CHO) are meta directing.
The choice of electrophile also dictates the outcome. Common electrophiles include:
- Nitration: Nitronium ion (NO2+) generated from nitric acid and sulfuric acid.
- Halogenation: Chlorine (Cl2) or bromine (Br2) in the presence of a Lewis acid catalyst like FeCl3 or AlBr3.
- Sulfonation: Sulfur trioxide (SO3) or fuming sulfuric acid.
- Friedel-Crafts Alkylation/Acylation: Alkyl halides or acid chlorides in the presence of a Lewis acid catalyst (e.g., AlCl3).
Careful consideration of the directing group and the desired regiochemistry is crucial in selecting the appropriate electrophile and conditions.
Functional Group Transformations: A Reagent-Centric Approach
Many organic synthesis strategies involve converting one functional group into another. This section explores common functional group interconversions and the reagents employed.
Oxidation and Reduction Reactions
Oxidation and reduction reactions are fundamental transformations in organic chemistry. Numerous reagents are available for these transformations, each with its own selectivity and limitations.
-
Oxidation of Alcohols: Primary alcohols can be oxidized to aldehydes or carboxylic acids, while secondary alcohols are oxidized to ketones. Common oxidizing agents include chromic acid (H2CrO4), potassium permanganate (KMnO4), and Jones reagent (CrO3 in H2SO4). More selective oxidizing agents like PCC (pyridinium chlorochromate) or Swern oxidation (DMSO, oxalyl chloride) can be employed to avoid over-oxidation.
-
Reduction of Ketones and Aldehydes: These are typically reduced to alcohols using reducing agents like lithium aluminum hydride (LiAlH4) or sodium borohydride (NaBH4). LiAlH4 is a more powerful reducing agent and can reduce esters and carboxylic acids, while NaBH4 is milder and more selective.
-
Reduction of Alkynes and Alkenes: Hydrogenation using catalysts like platinum, palladium, or nickel reduces alkynes to alkenes or alkanes. The selectivity can be controlled by the choice of catalyst and reaction conditions.
Protecting Groups: Safeguarding Functional Groups
Many organic molecules contain multiple functional groups. When performing a transformation on one functional group, it's often necessary to protect other reactive groups to avoid unwanted side reactions. Protecting groups are temporary modifications that mask the reactivity of a specific functional group. Their selection depends on the nature of the functional group to be protected and the reaction conditions. Common protecting groups include:
- Alcohols: tert-Butyldimethylsilyl (TBDMS) ether, benzyl ether (Bn), and acetyl (Ac) groups.
- Amines: Carbobenzyloxy (Cbz), tert-butoxycarbonyl (Boc), and benzyl (Bn) groups.
- Carbonyls: Acetals and ketals.
The choice of protecting group should consider its compatibility with subsequent reaction steps and its ease of removal.
Practical Considerations in Reagent Selection: Beyond the Theory
While understanding reaction mechanisms is crucial, several practical aspects need consideration:
-
Cost and Availability: Some reagents are expensive or difficult to obtain. Cost-effective alternatives should be considered, especially in large-scale syntheses.
-
Toxicity and Safety: Many reagents are toxic or hazardous. Appropriate safety precautions must be taken, including the use of personal protective equipment (PPE) and proper waste disposal.
-
Solvent Selection: The choice of solvent can significantly influence reaction rate, selectivity, and yield. Solvents should be chosen based on their polarity, ability to dissolve reactants, and compatibility with the reagents.
-
Reaction Conditions: Temperature, pressure, and reaction time all play a significant role in the outcome of a reaction. Optimizing these parameters is essential for achieving high yields and selectivity.
Case Studies: Applying Reagent Selection Principles
Let's examine a few specific synthetic transformations and the rationale behind the reagent choices.
Example 1: Conversion of a primary alcohol to an aldehyde
Converting a primary alcohol to an aldehyde requires a mild oxidizing agent to avoid over-oxidation to the carboxylic acid. Pyridinium chlorochromate (PCC) is a suitable reagent for this transformation due to its selectivity. Stronger oxidizing agents like chromic acid would lead to the formation of the carboxylic acid.
Example 2: Synthesis of an ether using Williamson ether synthesis
The Williamson ether synthesis involves the reaction of an alkoxide ion with an alkyl halide. The choice of alkyl halide is crucial; primary alkyl halides react faster via SN2 mechanisms. The alkoxide ion is typically generated by reacting an alcohol with a strong base like sodium hydride (NaH). The reaction is typically carried out in an aprotic solvent to avoid protonation of the alkoxide ion.
Example 3: Selective reduction of a ketone in the presence of an ester
Sodium borohydride (NaBH4) is a milder reducing agent compared to Lithium Aluminum Hydride (LiAlH4). While LiAlH4 can reduce both ketones and esters, NaBH4 selectively reduces ketones leaving esters untouched. This selectivity is crucial when the molecule contains both functional groups.
Conclusion: A Continuous Learning Process
Selecting the appropriate reagents for organic synthesis is a complex process that requires a thorough understanding of reaction mechanisms, reagent properties, and practical considerations. It's not merely about memorizing reagent-product pairs but rather about developing a problem-solving approach that integrates theoretical knowledge with practical expertise. Continuous learning, experimentation, and a deep understanding of chemical principles are vital for mastering this crucial aspect of organic synthesis. The field is constantly evolving with the development of new reagents and reaction methodologies, emphasizing the need for ongoing professional development for chemists working in this area. Mastering reagent selection is key to efficient, selective, and high-yielding organic synthesis.
Latest Posts
Related Post
Thank you for visiting our website which covers about Select The Appropriate Reagents To Achieve The Synthetic Transformation Shown . We hope the information provided has been useful to you. Feel free to contact us if you have any questions or need further assistance. See you next time and don't miss to bookmark.