Rank The Sequence Of Cross Bridge Cycling
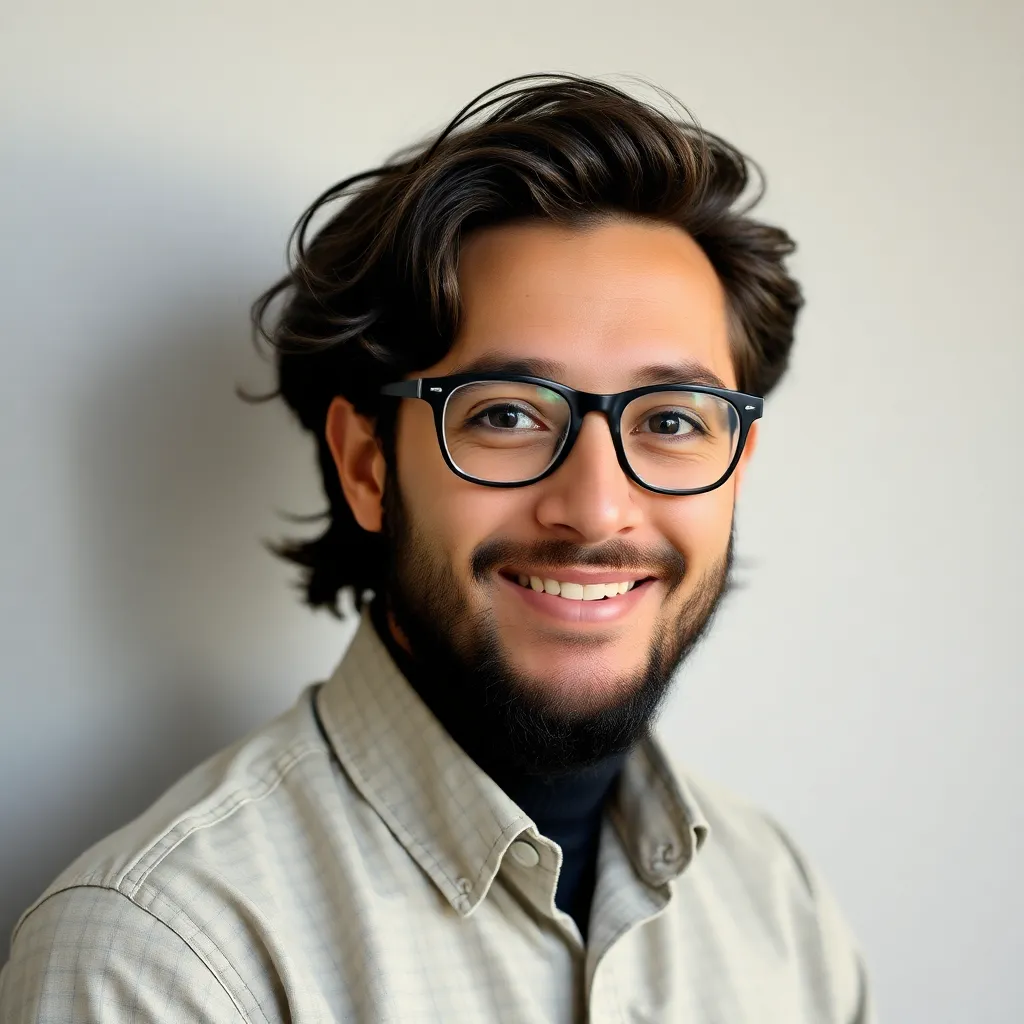
Holbox
May 13, 2025 · 6 min read
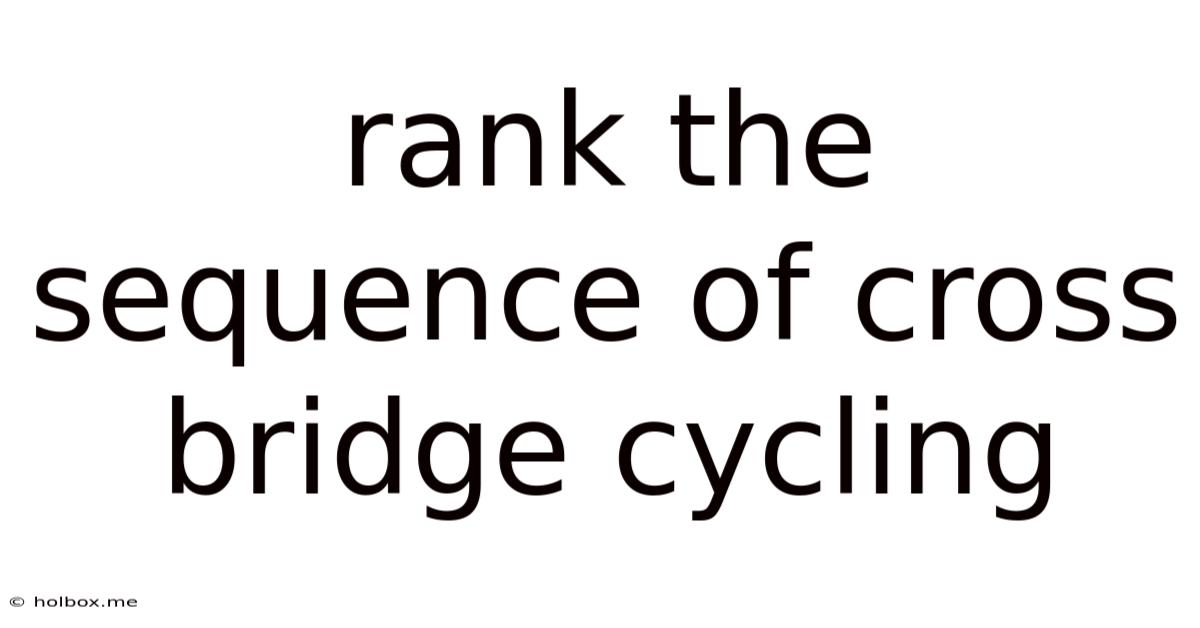
Table of Contents
- Rank The Sequence Of Cross Bridge Cycling
- Table of Contents
- Rank the Sequence of Cross-Bridge Cycling: A Deep Dive into Muscle Contraction
- The Key Players: Actin and Myosin
- Ranking the Cross-Bridge Cycling Sequence: A Step-by-Step Breakdown
- Deeper Dive into Each Stage: Molecular Mechanisms and Energetics
- Factors Influencing Cross-Bridge Cycling: Muscle Fiber Types and Metabolic Conditions
- Clinical Significance and Future Directions
- Conclusion: The Powerhouse of Movement
- Latest Posts
- Related Post
Rank the Sequence of Cross-Bridge Cycling: A Deep Dive into Muscle Contraction
Understanding the precise sequence of events in cross-bridge cycling is crucial to comprehending how muscles contract and generate force. This process, a complex interplay of biochemical reactions and structural changes, is the fundamental mechanism behind movement, posture, and countless other bodily functions. This article provides a detailed, ranked breakdown of the cross-bridge cycle, explaining each step and its importance in muscle physiology. We'll delve into the molecular players involved, the energetic requirements, and the factors influencing the overall efficiency of the process.
The Key Players: Actin and Myosin
Before diving into the ranked sequence, it's important to establish the central players: actin and myosin. These proteins are the fundamental building blocks of muscle fibers. Actin filaments, thin and intertwined, form the backbone of the I-band in a sarcomere (the functional unit of muscle). Myosin filaments, thick and adorned with myosin heads, reside primarily in the A-band. These myosin heads are the key actors in cross-bridge cycling, acting like tiny molecular motors.
Ranking the Cross-Bridge Cycling Sequence: A Step-by-Step Breakdown
The cross-bridge cycle is a cyclical process, repeatedly occurring to generate sustained muscle contraction. While the precise timing and duration of each step can vary depending on factors like muscle fiber type and metabolic conditions, the fundamental sequence remains consistent. Here's a ranked breakdown:
1. Attachment (ATP Hydrolysis): This is the initiating step. Myosin heads, in their high-energy conformation (due to ATP hydrolysis), bind to specific sites on the actin filament. This binding is highly specific and requires calcium ions (Ca²⁺). The release of inorganic phosphate (Pi) from the myosin head further strengthens the bond, initiating the power stroke.
2. Power Stroke: The most impactful step. Following the strong attachment, the myosin head undergoes a conformational change, pivoting and pulling the actin filament towards the center of the sarcomere. This "power stroke" is the actual force-generating event, shortening the sarcomere and leading to muscle contraction. ADP is released during this step.
3. Detachment (ATP Binding): To allow the cycle to continue, the myosin head must detach from the actin filament. This detachment is triggered by the binding of a new ATP molecule to the myosin head. This binding weakens the myosin-actin interaction, leading to dissociation.
4. Cocking (ATP Hydrolysis): The final stage before the cycle restarts. Once detached, the ATP molecule bound to the myosin head is hydrolyzed into ADP and Pi. This hydrolysis provides the energy required to "cock" the myosin head, returning it to its high-energy conformation, ready to initiate another cycle of attachment, power stroke, and detachment.
Deeper Dive into Each Stage: Molecular Mechanisms and Energetics
Let's explore each stage in more detail, focusing on the underlying molecular mechanisms and the role of energy:
1. Attachment: The Calcium Trigger:
- Calcium's Role: The initiation of cross-bridge cycling is exquisitely regulated by calcium ions (Ca²⁺). When a muscle is stimulated to contract, Ca²⁺ is released from the sarcoplasmic reticulum (SR), a specialized storage compartment within muscle cells.
- Troponin and Tropomyosin: Ca²⁺ binds to troponin, a protein complex associated with actin. This binding causes a conformational change in troponin, which then moves tropomyosin, another protein that usually blocks the myosin-binding sites on actin. This "unblocking" exposes the binding sites, allowing the myosin head to attach.
- ATP Hydrolysis and Pi Release: ATP hydrolysis by the myosin head provides the energy needed for the initial attachment and the subsequent power stroke. The release of inorganic phosphate (Pi) is a critical step, providing the "spring-loaded" state for the power stroke.
2. Power Stroke: The Force-Generating Event:
- Conformational Change: The power stroke involves a significant conformational change in the myosin head, pivoting it and pulling the actin filament. This movement is driven by the energy stored in the myosin head from previous ATP hydrolysis.
- Sliding Filament Theory: This power stroke is the fundamental mechanism underlying the sliding filament theory of muscle contraction, where actin and myosin filaments slide past each other, shortening the sarcomere.
- ADP Release: ADP is released during the power stroke, further strengthening the myosin-actin bond and maximizing force generation.
3. Detachment: The ATP-Driven Dissociation:
- ATP Binding: The binding of a new ATP molecule to the myosin head is crucial for detachment. This binding causes a conformational change in the myosin head, reducing its affinity for actin.
- Releasing the Grip: The attachment between the myosin head and the actin filament is broken, allowing the myosin head to detach and prepare for the next cycle.
- Reversibility: This detachment step is essential for the reversibility of the cycle. Without it, the muscle would remain rigidly contracted.
4. Cocking: The Energy Reset:
- ATP Hydrolysis: ATP hydrolysis fuels the "cocking" of the myosin head, returning it to its high-energy conformation.
- Energy for the Next Cycle: This step resets the myosin head, preparing it for the next round of attachment, power stroke, and detachment, ensuring the continuation of the cyclical process.
- Phosphorylation and Dephosphorylation: This process showcases the crucial role of phosphorylation (addition of a phosphate group) and dephosphorylation (removal of a phosphate group) in regulating myosin head conformation and energy state.
Factors Influencing Cross-Bridge Cycling: Muscle Fiber Types and Metabolic Conditions
Several factors can modulate the rate and efficiency of cross-bridge cycling. These include:
-
Muscle Fiber Type: Different muscle fiber types (e.g., slow-twitch, fast-twitch) have distinct characteristics that influence the speed and duration of cross-bridge cycling. Slow-twitch fibers, rich in mitochondria, utilize oxidative metabolism and sustain contractions for longer periods. Fast-twitch fibers, on the other hand, rely more on glycolysis and are capable of rapid, powerful contractions.
-
ATP Availability: The availability of ATP is paramount. A lack of ATP can lead to rigor mortis, a state where muscles remain rigidly contracted due to the inability of myosin heads to detach from actin.
-
Calcium Concentration: The concentration of Ca²⁺ in the cytosol precisely regulates the rate of cross-bridge cycling. Higher Ca²⁺ levels lead to more cross-bridges forming and a stronger contraction.
-
Temperature: Temperature affects the rate of enzyme-catalyzed reactions, including ATP hydrolysis. Higher temperatures initially increase the rate of cross-bridge cycling but can lead to denaturation of proteins at excessively high temperatures.
-
Muscle Length: The optimal length of the sarcomere influences the number of cross-bridges that can form. At overly stretched or compressed lengths, fewer cross-bridges can form, resulting in reduced force generation.
Clinical Significance and Future Directions
A thorough understanding of cross-bridge cycling is crucial in various clinical settings. Conditions like muscular dystrophy, myasthenia gravis, and various muscle injuries often involve disruptions in the cross-bridge cycle, leading to muscle weakness or dysfunction. Research into the molecular mechanisms underlying these disruptions is vital for developing effective treatments. Future research directions include exploring the detailed mechanisms of regulation of cross-bridge cycling at the single-molecule level, further elucidating the role of various isoforms of myosin and actin, and developing novel therapeutic strategies targeting specific steps in the cycle to address muscle-related diseases.
Conclusion: The Powerhouse of Movement
The cross-bridge cycle is a remarkable example of biological precision and efficiency. Its intricate steps, regulated by a complex interplay of proteins and ions, are the driving force behind muscle contraction and movement. By understanding the ranked sequence of events and the underlying molecular mechanisms, we can appreciate the fundamental process that enables us to walk, run, lift, and perform a myriad of other actions that are essential to life. Further research into this fascinating area will undoubtedly continue to unlock new insights into muscle physiology and provide avenues for treating muscle-related diseases.
Latest Posts
Related Post
Thank you for visiting our website which covers about Rank The Sequence Of Cross Bridge Cycling . We hope the information provided has been useful to you. Feel free to contact us if you have any questions or need further assistance. See you next time and don't miss to bookmark.