Radioactive Decay Is Likely To Occur When
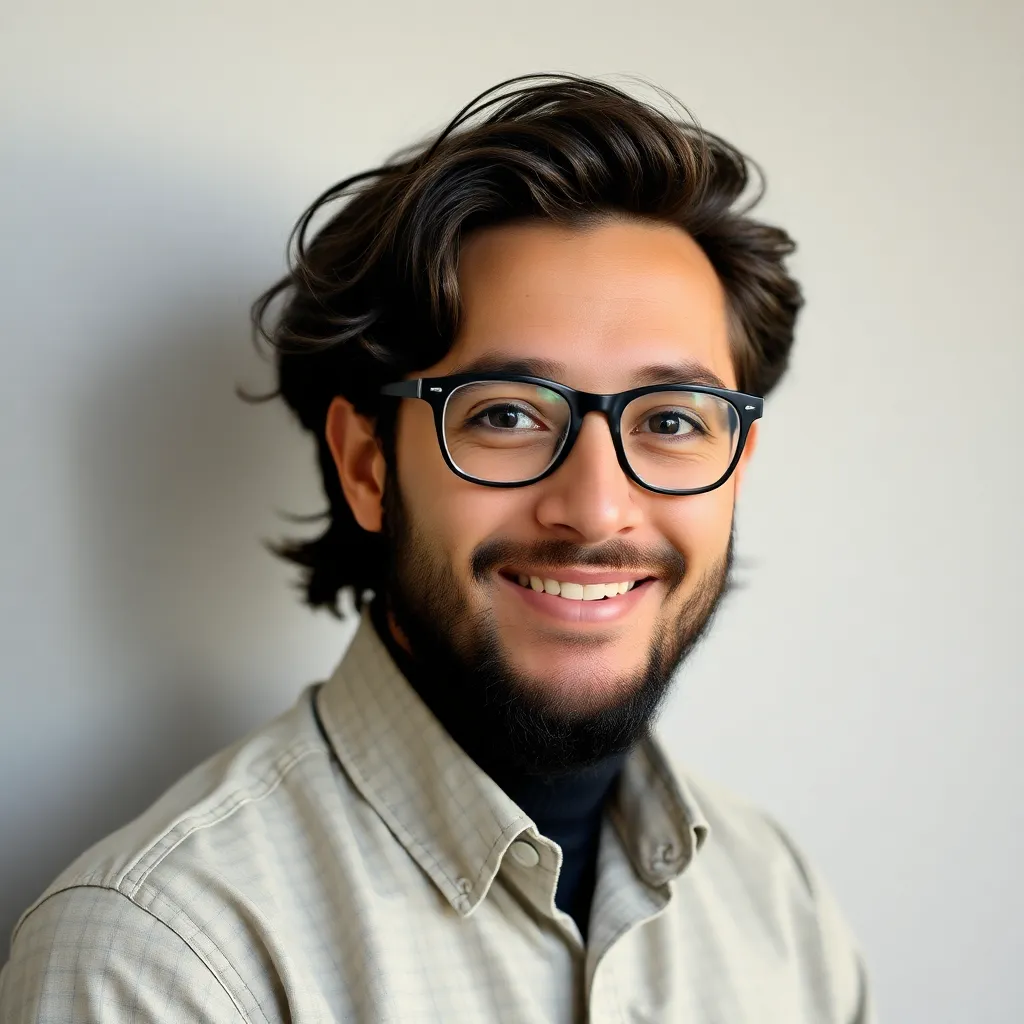
Holbox
Apr 12, 2025 · 7 min read

Table of Contents
- Radioactive Decay Is Likely To Occur When
- Table of Contents
- Radioactive Decay: When and Why It Happens
- Understanding Atomic Nuclei
- The Neutron-to-Proton Ratio: A Key Factor
- Consequences of Imbalance:
- Other Factors Influencing Radioactive Decay
- 1. Excited Nuclear States:
- 2. Nuclear Shell Structure:
- 3. Nuclear Isomerism:
- Types of Radioactive Decay
- Predicting Radioactive Decay: Half-life
- Applications of Radioactive Decay
- Conclusion
- Latest Posts
- Latest Posts
- Related Post
Radioactive Decay: When and Why It Happens
Radioactive decay, a fundamental process in nuclear physics, is the spontaneous transformation of an unstable atomic nucleus into a more stable one. This transformation releases energy in the form of ionizing radiation, altering the nucleus's composition. Understanding the conditions that favor radioactive decay is crucial in various fields, from nuclear medicine to geology. This article delves into the intricacies of radioactive decay, exploring the factors that make it likely to occur.
Understanding Atomic Nuclei
Before delving into the conditions favoring radioactive decay, let's establish a basic understanding of atomic nuclei. An atomic nucleus consists of protons and neutrons, collectively called nucleons. The number of protons defines the element (atomic number, Z), while the total number of protons and neutrons determines the mass number (A). Isotopes are atoms of the same element with different numbers of neutrons, thus having the same Z but different A.
The stability of an atomic nucleus is determined by the delicate balance between the strong nuclear force, which binds protons and neutrons together, and the electromagnetic force, which repels protons due to their positive charge. This balance is significantly influenced by the neutron-to-proton ratio (N/Z).
The Neutron-to-Proton Ratio: A Key Factor
The neutron-to-proton ratio (N/Z) is a critical determinant of nuclear stability. For lighter elements (Z < 20), a stable nucleus typically has an N/Z ratio close to 1. However, as the atomic number increases, the required N/Z ratio for stability gradually increases to maintain the balance against the increasing proton-proton repulsion. Nuclei with N/Z ratios significantly deviating from this optimal value are inherently unstable and prone to radioactive decay.
Consequences of Imbalance:
-
Neutron-rich nuclei (N/Z > 1): These nuclei have an excess of neutrons, leading to instability. They are likely to undergo beta-minus decay (β⁻ decay), where a neutron transforms into a proton, an electron (β⁻ particle), and an antineutrino. This process reduces the number of neutrons and increases the number of protons, moving the N/Z ratio closer to the stability zone.
-
Proton-rich nuclei (N/Z < 1): These nuclei have a deficiency of neutrons relative to protons. They are prone to several decay modes, including beta-plus decay (β⁺ decay) or electron capture. In β⁺ decay, a proton transforms into a neutron, a positron (β⁺ particle), and a neutrino. Electron capture involves the nucleus absorbing an inner-shell electron, converting a proton to a neutron and emitting a neutrino. Both processes increase the number of neutrons and decrease the number of protons, adjusting the N/Z ratio towards stability.
-
Nuclei with high atomic numbers (Z > 83): Even with an optimal N/Z ratio, extremely heavy nuclei (those with Z > 83) are inherently unstable due to the overwhelming strength of the electromagnetic repulsion between the large number of protons. These nuclei typically undergo alpha decay, emitting an alpha particle (a helium nucleus consisting of two protons and two neutrons). Alpha decay reduces both the number of protons and neutrons, significantly decreasing the size and instability of the nucleus.
Other Factors Influencing Radioactive Decay
While the N/Z ratio is the dominant factor, other factors can influence the likelihood of radioactive decay:
1. Excited Nuclear States:
Atomic nuclei can exist in various energy levels, analogous to electrons in atoms. When a nucleus is in an excited state (higher energy level), it's inherently less stable and more likely to decay to a lower energy state by emitting gamma rays (γ rays), a form of electromagnetic radiation. This transition doesn't change the number of protons or neutrons, only the energy state of the nucleus.
2. Nuclear Shell Structure:
Similar to electron shells in atoms, nucleons (protons and neutrons) are arranged in energy levels or shells within the nucleus. Nuclei with completely filled shells (magic numbers of protons or neutrons: 2, 8, 20, 28, 50, 82, 126) are exceptionally stable. Nuclei with nucleon numbers close to these magic numbers exhibit enhanced stability compared to their neighbors. Conversely, nuclei far from magic numbers are more prone to decay.
3. Nuclear Isomerism:
Nuclear isomers are isotopes with the same number of protons and neutrons but different energy states. They represent excited states of a nucleus that are relatively long-lived (metastable). These metastable isomers can undergo isomeric transition, decaying to a lower energy state by emitting gamma rays.
Types of Radioactive Decay
Several types of radioactive decay exist, each characterized by the emitted particle or radiation:
-
Alpha Decay (α decay): Emission of an alpha particle (²He), reducing the atomic number by 2 and the mass number by 4. Common in heavy nuclei.
-
Beta-minus Decay (β⁻ decay): Emission of a beta-minus particle (electron) and an antineutrino. A neutron transforms into a proton, increasing the atomic number by 1 while the mass number remains constant.
-
Beta-plus Decay (β⁺ decay): Emission of a beta-plus particle (positron) and a neutrino. A proton transforms into a neutron, decreasing the atomic number by 1 while the mass number remains constant.
-
Electron Capture (EC): The nucleus captures an inner-shell electron, converting a proton to a neutron. A neutrino is emitted. The atomic number decreases by 1, while the mass number remains constant.
-
Gamma Decay (γ decay): Emission of a gamma ray (high-energy photon), resulting from a transition of the nucleus from a higher energy state to a lower energy state. The atomic number and mass number remain unchanged.
-
Spontaneous Fission: The nucleus splits into two or more smaller nuclei, typically accompanied by the release of neutrons and significant energy. This is common in very heavy nuclei.
Predicting Radioactive Decay: Half-life
The rate at which a radioactive isotope decays is characterized by its half-life (t₁/₂), which is the time required for half of the nuclei in a sample to decay. Half-lives range from fractions of a second to billions of years, depending on the isotope and its stability. A shorter half-life indicates a higher decay rate, meaning the isotope is more unstable.
The decay process follows first-order kinetics, meaning the decay rate is proportional to the number of radioactive nuclei present. This is described mathematically by the equation:
N(t) = N₀ * e^(-λt)
Where:
- N(t) is the number of radioactive nuclei remaining at time t
- N₀ is the initial number of radioactive nuclei
- λ is the decay constant (related to the half-life)
- e is the base of the natural logarithm
Applications of Radioactive Decay
Understanding radioactive decay has numerous applications across various fields:
-
Nuclear Medicine: Radioactive isotopes are used for diagnosis and treatment of diseases. For instance, certain isotopes emit gamma rays used in imaging techniques like PET (Positron Emission Tomography) scans. Other isotopes are used in radiotherapy to target and destroy cancerous cells.
-
Radioactive Dating: Radioactive isotopes with known half-lives are used to determine the age of geological formations, artifacts, and other materials. Carbon-14 dating is a widely used technique for dating organic materials.
-
Nuclear Power Generation: Nuclear reactors utilize controlled nuclear fission (a type of radioactive decay) to generate electricity.
-
Geological Studies: The presence and distribution of radioactive isotopes in rocks and minerals provide insights into the Earth's geological history and processes.
-
Industrial Applications: Radioactive isotopes find applications in various industrial processes, such as gauging thickness, detecting leaks, and sterilizing medical equipment.
Conclusion
Radioactive decay is a complex yet fundamental process governed primarily by the neutron-to-proton ratio within the atomic nucleus. Other factors, including excited nuclear states, nuclear shell structure, and nuclear isomerism, also influence the likelihood of decay. The various types of radioactive decay—alpha, beta, gamma, and spontaneous fission—each represent different pathways towards nuclear stability. Understanding these factors and the kinetics of radioactive decay is essential in numerous scientific and technological applications, impacting fields ranging from medicine to geology and energy production. Further research continually refines our understanding of this fascinating and crucial process within the realm of nuclear physics.
Latest Posts
Latest Posts
-
Depending On The Incident Size And Complexity
Apr 16, 2025
-
Chevelle Inc Has Sales Of 39500
Apr 16, 2025
-
Construct A Three Step Synthesis Of 1 2 Epoxycyclopentane From Bromocyclopentane
Apr 16, 2025
-
Infants And Children Prenatal Through Middle Childhood
Apr 16, 2025
-
10 Painters Can Paint A Building In 16 Hours
Apr 16, 2025
Related Post
Thank you for visiting our website which covers about Radioactive Decay Is Likely To Occur When . We hope the information provided has been useful to you. Feel free to contact us if you have any questions or need further assistance. See you next time and don't miss to bookmark.