Predict The Major Product Of The Following Reaction Sequence
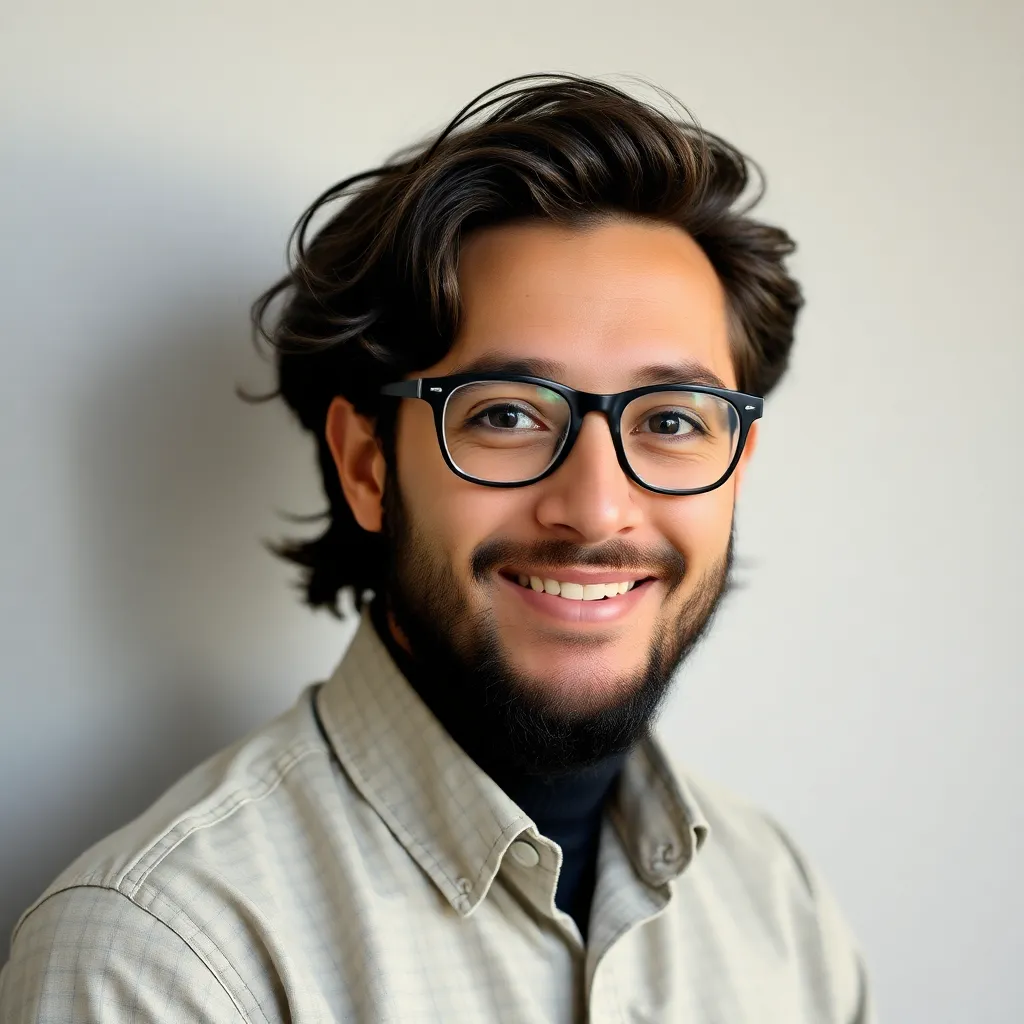
Holbox
Apr 04, 2025 · 6 min read

Table of Contents
- Predict The Major Product Of The Following Reaction Sequence
- Table of Contents
- Predicting the Major Product: A Deep Dive into Reaction Sequences
- Understanding Reaction Mechanisms: The Foundation of Prediction
- Common Reaction Types and Their Predictive Power
- Factors Influencing Product Prediction: Steric Hindrance and Electronic Effects
- Predicting Products in Multi-Step Reactions
- Advanced Techniques and Considerations: Spectroscopy and Computational Chemistry
- Examples of Predicting Major Products in Reaction Sequences
- Conclusion: Mastering the Art of Prediction
- Latest Posts
- Latest Posts
- Related Post
Predicting the Major Product: A Deep Dive into Reaction Sequences
Predicting the major product of a reaction sequence is a cornerstone of organic chemistry. It requires a thorough understanding of reaction mechanisms, functional group transformations, and the principles of regio- and stereoselectivity. This article will explore various strategies for accurately predicting the major product, focusing on common reaction types and providing detailed examples. We'll delve into the nuances of predicting outcomes, emphasizing the importance of considering steric hindrance, electronic effects, and reaction conditions.
Understanding Reaction Mechanisms: The Foundation of Prediction
Before attempting to predict the major product of any reaction sequence, it's crucial to understand the underlying reaction mechanism. This mechanistic understanding allows us to predict the intermediate species formed and ultimately, the final product. Knowing the mechanism allows us to anticipate the regioselectivity (preference for a specific position on a molecule) and stereoselectivity (preference for a specific spatial arrangement of atoms) of the reaction.
For example, consider the SN1 and SN2 mechanisms. SN1 reactions proceed through a carbocation intermediate, making them susceptible to carbocation rearrangements and leading to a mixture of products. In contrast, SN2 reactions involve a concerted mechanism, resulting in inversion of stereochemistry at the reaction center. Understanding these differences is critical in predicting the outcome.
Common Reaction Types and Their Predictive Power
Several reaction types are frequently encountered in organic chemistry. Mastering their mechanisms and predicting their products is essential for success. Let's explore some key reactions:
1. Electrophilic Aromatic Substitution: This reaction involves the substitution of an electrophile for a hydrogen atom on an aromatic ring. The position of substitution is determined by the directing effects of the substituents already present on the ring. Activating groups (e.g., -OH, -NH2) direct the electrophile to the ortho and para positions, while deactivating groups (e.g., -NO2, -COOH) direct the electrophile to the meta position.
Example: Nitration of toluene. The methyl group is an activating, ortho/para-directing group. Therefore, the major product will be a mixture of ortho- and para-nitrotoluene, with the para isomer often being the major component due to less steric hindrance.
2. Nucleophilic Acyl Substitution: This reaction involves the substitution of a leaving group on an acyl group (e.g., carbonyl group) by a nucleophile. The mechanism involves a tetrahedral intermediate, which then collapses to form the final product. The reactivity of acyl derivatives follows the order: acyl chloride > anhydride > ester > amide.
Example: Hydrolysis of an ester. The nucleophile (water) attacks the carbonyl carbon, forming a tetrahedral intermediate. This intermediate then collapses, resulting in the formation of a carboxylic acid and an alcohol.
3. Addition Reactions: These reactions involve the addition of two or more atoms or groups to a multiple bond (e.g., C=C, C≡C). The regioselectivity of addition reactions is often governed by Markovnikov's rule, which states that the electrophile adds to the carbon atom with the most hydrogen atoms. Stereoselectivity can also be observed, with syn or anti addition being possible depending on the mechanism.
Example: Addition of HBr to propene. According to Markovnikov's rule, the hydrogen atom adds to the carbon atom with more hydrogens, resulting in 2-bromopropane as the major product.
4. Elimination Reactions: These reactions involve the removal of two atoms or groups from adjacent carbon atoms, resulting in the formation of a multiple bond. The regioselectivity and stereoselectivity of elimination reactions depend on the substrate, the base, and the reaction conditions. Zaitsev's rule states that the major product is the alkene with the most substituted double bond.
Example: Dehydration of 2-butanol. This reaction forms a mixture of but-1-ene and but-2-ene, with but-2-ene being the major product due to Zaitsev's rule.
5. Grignard Reactions: Grignard reagents (RMgX) are powerful nucleophiles that react with a variety of electrophiles, including carbonyl compounds. They add to the carbonyl group, forming a new carbon-carbon bond. The product is typically an alcohol after acid workup.
Example: Reaction of a Grignard reagent with formaldehyde. This reaction leads to the formation of a primary alcohol after acid workup.
Factors Influencing Product Prediction: Steric Hindrance and Electronic Effects
Several factors can significantly influence the major product formed in a reaction sequence.
1. Steric Hindrance: Bulky groups can hinder the approach of reactants, affecting reaction rates and product distribution. In SN2 reactions, for instance, steric hindrance at the reaction center significantly reduces the reaction rate.
2. Electronic Effects: The electronic properties of substituents can influence the reactivity of a molecule. Electron-donating groups increase electron density at a particular site, making it more susceptible to electrophilic attack. Conversely, electron-withdrawing groups decrease electron density, reducing reactivity.
3. Reaction Conditions: Factors like temperature, solvent, and the concentration of reactants can greatly impact the outcome of a reaction. For example, high temperatures often favor elimination reactions over substitution reactions. The solvent can influence the stability of intermediates, thereby affecting the product distribution.
Predicting Products in Multi-Step Reactions
Predicting products in multi-step reactions requires a systematic approach. It's crucial to carefully consider the outcome of each step individually before moving to the next. Each intermediate product will affect the subsequent reactions, often leading to complex product formation. The prediction process becomes a step-by-step analysis of transformations.
Advanced Techniques and Considerations: Spectroscopy and Computational Chemistry
Advanced techniques like NMR spectroscopy and mass spectrometry are invaluable in identifying and confirming the structures of reaction products. Computational chemistry methods, such as density functional theory (DFT) calculations, can be used to predict reaction pathways and product stability. These tools enhance predictive power, especially for complex reactions where intuition may not suffice.
Examples of Predicting Major Products in Reaction Sequences
Let's illustrate product prediction with a couple of detailed examples:
Example 1:
A reaction sequence might involve the bromination of an alkene followed by an SN2 reaction with a nucleophile. The first step, bromination, adds bromine across the double bond. The regiochemistry is determined by the electrophilic addition mechanism, typically following Markovnikov's rule if there is a difference in substitution around the double bond. The stereochemistry might be anti-addition or syn-addition depending on the mechanism (e.g., bromonium ion intermediate).
The second step, SN2, would involve nucleophilic attack at the carbon bearing the bromine atom, resulting in inversion of stereochemistry at that carbon. Careful analysis of these steps, along with consideration of steric effects, will lead to the accurate prediction of the major product.
Example 2: A multi-step synthesis may start with an aromatic compound undergoing nitration, followed by reduction to an amine, and then a diazotization reaction to synthesize a diazonium salt. Each step presents its own challenges in prediction – for example, the nitration step's regioselectivity depends on the existing substituents, the reduction must account for any protecting groups, and diazotization is sensitive to reaction conditions. Each step needs to be examined thoroughly.
Conclusion: Mastering the Art of Prediction
Predicting the major product of a reaction sequence is a crucial skill in organic chemistry. It requires a solid understanding of reaction mechanisms, functional group transformations, and the influence of steric and electronic factors. A systematic, step-by-step approach, combined with knowledge of common reaction types and the application of advanced techniques when necessary, is essential for accurately determining the major product. With practice and a keen eye for detail, one can master this art and efficiently navigate the complex world of organic reaction sequences. Remember, the accuracy of prediction improves with a strong grasp of fundamental principles and a deep understanding of the intricate interplay of reaction conditions and molecular structure.
Latest Posts
Latest Posts
-
Following Is Not A Security Standard
Apr 15, 2025
-
Consider The Lewis Structure Shown For Thionitromethane
Apr 15, 2025
-
For What Organization Did Jenny Holzer Design T Shirts
Apr 15, 2025
-
Within The Relevant Range Of Activity
Apr 15, 2025
-
Leadership Roles And Management Functions In Nursing Theory And Application
Apr 15, 2025
Related Post
Thank you for visiting our website which covers about Predict The Major Product Of The Following Reaction Sequence . We hope the information provided has been useful to you. Feel free to contact us if you have any questions or need further assistance. See you next time and don't miss to bookmark.