Place The Steps Of Eukaryotic Dna Replication In Order
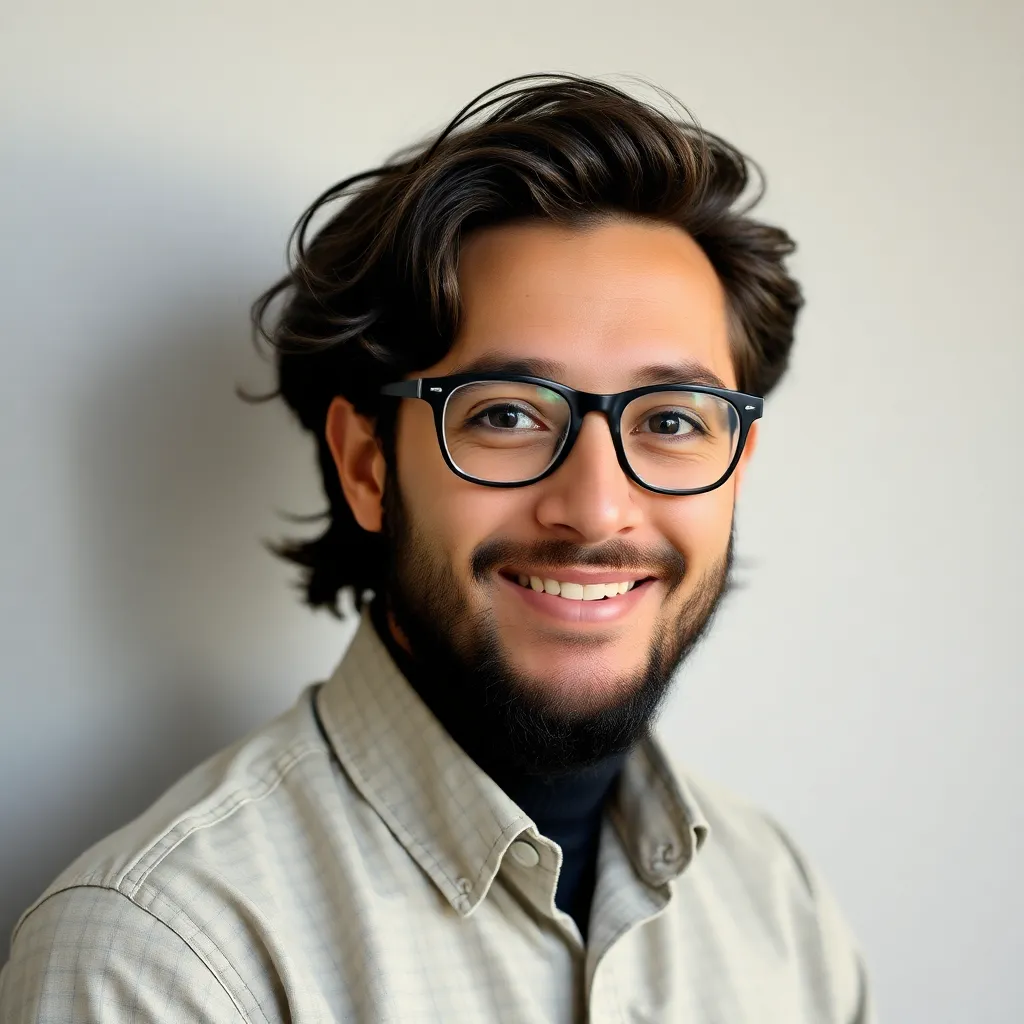
Holbox
May 08, 2025 · 6 min read
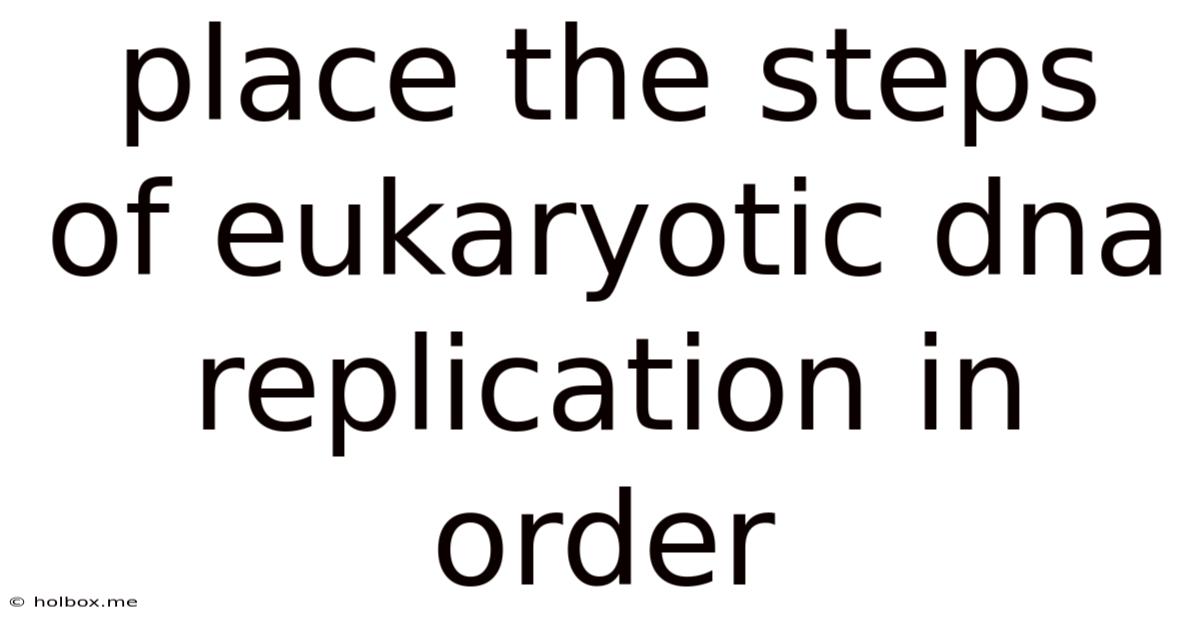
Table of Contents
- Place The Steps Of Eukaryotic Dna Replication In Order
- Table of Contents
- The Eukaryotic DNA Replication Process: A Step-by-Step Guide
- Step 1: Initiation – Identifying the Origin of Replication
- Key Players in Initiation:
- Step 2: Unwinding the DNA Double Helix – The Role of Helicases
- Key Enzymes in Unwinding:
- Step 3: Primer Synthesis – Getting Started with DNA Synthesis
- Significance of RNA Primers:
- Step 4: Elongation – The DNA Polymerase Machinery
- Leading vs. Lagging Strand Synthesis:
- Step 5: Proofreading and Error Correction – Maintaining Fidelity
- Mechanisms of Error Correction:
- Step 6: Termination – Completing Replication
- Key Processes in Termination:
- Step 7: Post-Replicative Processes – Ensuring Genomic Stability
- Understanding the Interplay: A Holistic View
- Latest Posts
- Related Post
The Eukaryotic DNA Replication Process: A Step-by-Step Guide
Eukaryotic DNA replication, a fundamental process in cell biology, is far more complex than its prokaryotic counterpart. The sheer size and linear nature of eukaryotic chromosomes, along with the intricate organization of chromatin, necessitate a tightly regulated and multi-step process. This detailed guide will walk you through the stages of eukaryotic DNA replication, providing insights into the key players and mechanisms involved. Understanding this process is crucial for comprehending numerous biological phenomena, including cell growth, development, and disease.
Step 1: Initiation – Identifying the Origin of Replication
Unlike prokaryotes with a single origin of replication, eukaryotic genomes possess multiple origins of replication scattered throughout their chromosomes. This is essential to ensure timely completion of DNA replication within the relatively short timeframe of the S phase of the cell cycle. The precise location of these origins isn't completely random; certain DNA sequences, termed replication origins, are preferentially selected.
Key Players in Initiation:
- Origin Recognition Complex (ORC): This protein complex binds to replication origins throughout the cell cycle, marking them as potential sites for initiation.
- Licensing Factors: These proteins, including Cdt1 and Cdc6, are crucial for preparing origins for replication. They load Mini-chromosome maintenance (MCM) proteins onto the DNA, forming the pre-replicative complex (pre-RC). This complex acts as a key licensing factor, ensuring that DNA replication only occurs once per cell cycle.
- Cyclin-Dependent Kinases (CDKs): These enzymes, active during S phase, trigger the activation of the pre-RC, initiating DNA unwinding and recruitment of other replication machinery. Their controlled activation is critical for coordinating DNA replication with the cell cycle.
The assembly of the pre-RC is a tightly regulated process. Its formation is restricted to the G1 phase of the cell cycle, preventing re-replication within a single cycle. This regulation involves the controlled synthesis and degradation of licensing factors.
Step 2: Unwinding the DNA Double Helix – The Role of Helicases
Once the pre-RC is activated, DNA unwinding begins. This is primarily achieved by DNA helicases, motor proteins that use ATP hydrolysis to separate the two DNA strands, creating a replication fork. The unwinding process generates torsional stress ahead of the replication fork, which is relieved by topoisomerases. These enzymes cut and rejoin the DNA strands, relieving the strain and preventing the formation of supercoils.
Key Enzymes in Unwinding:
- DNA Helicase: The major enzyme responsible for separating the DNA strands. Several types of helicases are involved in eukaryotic replication, each with distinct roles and regulation.
- Single-Strand Binding Proteins (SSBs): These proteins coat the separated DNA strands, preventing them from re-annealing and protecting them from nuclease degradation. They also provide a stable template for DNA polymerase.
- Topoisomerases: These enzymes prevent the build-up of torsional stress during unwinding. Type I topoisomerases cut and rejoin a single strand of DNA, while Type II topoisomerases cut both strands.
Step 3: Primer Synthesis – Getting Started with DNA Synthesis
DNA polymerases cannot initiate DNA synthesis de novo; they require a pre-existing 3'-OH group to add nucleotides. This is provided by RNA primers, synthesized by the enzyme primase. Primase is a type of RNA polymerase that synthesizes short RNA sequences complementary to the DNA template. These RNA primers provide the starting point for DNA polymerase to begin synthesizing new DNA strands.
Significance of RNA Primers:
- Initiating DNA synthesis: Provide the essential 3'-OH group for DNA polymerase.
- Leading and lagging strand synthesis: Multiple primers are required for lagging strand synthesis, due to its discontinuous nature.
- Primer removal: The RNA primers are subsequently removed and replaced with DNA by DNA polymerase δ (delta) and DNA polymerase ε (epsilon).
Step 4: Elongation – The DNA Polymerase Machinery
Elongation is the stage where the bulk of new DNA is synthesized. Two major DNA polymerases are involved:
- DNA polymerase α (alpha): A complex that includes primase and has a low processivity. It initiates DNA synthesis by extending the RNA primers.
- DNA polymerase δ (delta): The primary polymerase responsible for lagging strand synthesis. High processivity ensures efficient and rapid synthesis.
- DNA polymerase ε (epsilon): The primary polymerase responsible for leading strand synthesis. High processivity ensures efficient and rapid synthesis.
These polymerases work in conjunction with other proteins to ensure accurate and efficient DNA replication. The sliding clamp, a protein ring that encircles the DNA, enhances the processivity of DNA polymerases, preventing them from dissociating from the DNA template. Clamp loaders load the sliding clamp onto the DNA.
Leading vs. Lagging Strand Synthesis:
The leading strand is synthesized continuously in the 5' to 3' direction, following the replication fork. The lagging strand, however, is synthesized discontinuously in short fragments called Okazaki fragments. Each Okazaki fragment requires its own RNA primer.
Step 5: Proofreading and Error Correction – Maintaining Fidelity
DNA polymerases possess a remarkable ability to proofread their work. They have a 3' to 5' exonuclease activity that allows them to remove incorrectly incorporated nucleotides. This proofreading mechanism significantly reduces the error rate during DNA replication, maintaining the fidelity of the genome.
Mechanisms of Error Correction:
- 3' to 5' exonuclease activity: Removes mismatched nucleotides.
- Mismatch repair (MMR): A post-replication repair system that identifies and corrects mismatched base pairs that escape proofreading.
- Base excision repair (BER): Removes and replaces damaged or modified bases.
Step 6: Termination – Completing Replication
Termination of DNA replication involves the completion of lagging strand synthesis, removal of RNA primers, and ligation of Okazaki fragments. The final step involves resolving the remaining gaps and ensuring the integrity of the newly synthesized DNA.
Key Processes in Termination:
- Removal of RNA primers: RNA primers are removed by RNase H and flap endonuclease.
- Filling gaps: The gaps left by primer removal are filled by DNA polymerases.
- Ligation: Okazaki fragments are joined together by DNA ligase, forming a continuous DNA strand.
- Telomere Replication: The ends of linear chromosomes, called telomeres, pose a unique challenge for replication, as the lagging strand cannot be completely replicated. The enzyme telomerase extends telomeres, preventing chromosome shortening.
Step 7: Post-Replicative Processes – Ensuring Genomic Stability
Following replication, several post-replicative processes are essential for maintaining genomic stability. These include:
- Chromatin assembly: New histones are incorporated into the newly synthesized DNA, recreating the chromatin structure.
- DNA repair: Remaining DNA damage is repaired by various repair pathways.
- Cell cycle checkpoints: Checkpoints monitor the fidelity of DNA replication and ensure that any errors are corrected before the cell proceeds to mitosis.
Understanding the Interplay: A Holistic View
The entire process of eukaryotic DNA replication is a tightly orchestrated symphony of numerous proteins working in concert. The precise timing and regulation of each step are critical for accurate and efficient DNA duplication. Disruptions in any of these steps can lead to mutations, genomic instability, and potentially, disease. Further research continues to unveil the intricate details of this fascinating process, providing a deeper understanding of life itself.
This comprehensive guide provides a detailed overview of eukaryotic DNA replication. Remember to consult further scientific literature for an even deeper dive into specific aspects of this complex and dynamic process. The accuracy and efficiency of DNA replication are essential for maintaining the integrity of the genome and ensuring the healthy functioning of cells and organisms. Understanding this process is fundamental to comprehending many areas of biology, including genetics, cell biology, and medicine.
Latest Posts
Related Post
Thank you for visiting our website which covers about Place The Steps Of Eukaryotic Dna Replication In Order . We hope the information provided has been useful to you. Feel free to contact us if you have any questions or need further assistance. See you next time and don't miss to bookmark.