Place The Events Of Synaptic Transmission In Order
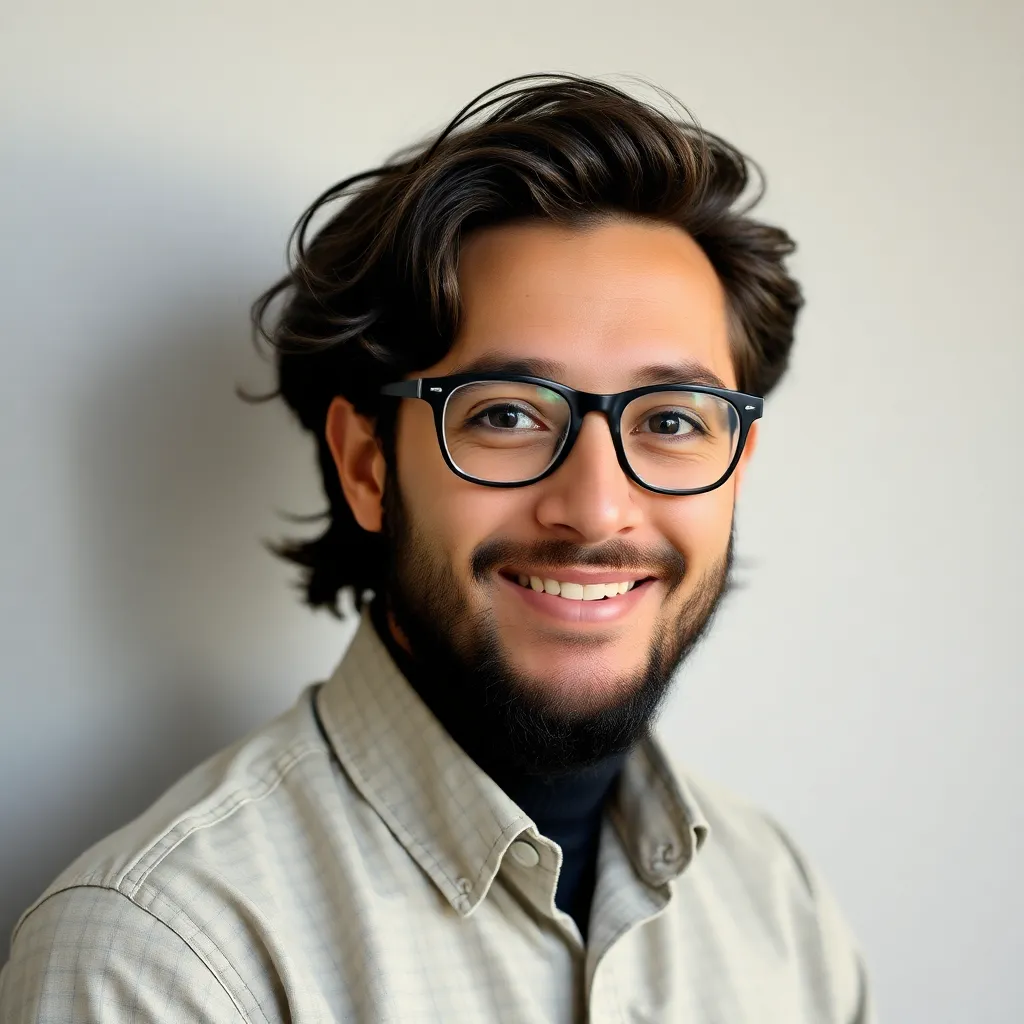
Holbox
Apr 07, 2025 · 6 min read

Table of Contents
- Place The Events Of Synaptic Transmission In Order
- Table of Contents
- Placing the Events of Synaptic Transmission in Order: A Comprehensive Guide
- The Players: A Cast of Thousands (of Molecules)
- The Sequence of Events: A Step-by-Step Guide
- The Summation of Postsynaptic Potentials: A Balancing Act
- Modulation of Synaptic Transmission: Fine-Tuning the Signal
- Clinical Significance: When Synaptic Transmission Goes Wrong
- Conclusion: A Complex and Dynamic Process
- Latest Posts
- Latest Posts
- Related Post
Placing the Events of Synaptic Transmission in Order: A Comprehensive Guide
Synaptic transmission, the process by which neurons communicate with each other and with other cells, is a fundamental process in the nervous system. Understanding the precise order of events involved is crucial for grasping how the brain functions, how diseases affect neural communication, and how drugs can influence behaviour. This article will provide a detailed, step-by-step breakdown of synaptic transmission, focusing on chemical synapses – the most common type. We'll explore each stage, highlighting key players and mechanisms, using clear language and illustrative examples.
The Players: A Cast of Thousands (of Molecules)
Before diving into the sequential events, let's briefly introduce the key players. Synaptic transmission hinges on the interaction of several crucial components:
- Presynaptic Neuron: The neuron sending the signal. It contains synaptic vesicles filled with neurotransmitters.
- Postsynaptic Neuron: The neuron receiving the signal. It possesses receptors specific to the neurotransmitter released by the presynaptic neuron.
- Synaptic Cleft: The narrow gap (approximately 20-40 nanometers) separating the presynaptic and postsynaptic neurons. This gap is where the magic of neurotransmission happens.
- Neurotransmitters: Chemical messengers stored in synaptic vesicles. Examples include acetylcholine, dopamine, serotonin, glutamate, and GABA. Different neurotransmitters have different effects on postsynaptic neurons.
- Synaptic Vesicles: Small membrane-bound sacs within the presynaptic terminal, storing neurotransmitters.
- Receptors: Protein molecules located on the postsynaptic membrane. They bind to specific neurotransmitters, initiating a cellular response.
- Ion Channels: Protein pores in the neuronal membranes that allow specific ions (like sodium, potassium, chloride, and calcium) to pass through, creating electrical signals.
- Enzymes: Proteins that break down neurotransmitters in the synaptic cleft, terminating the signal.
The Sequence of Events: A Step-by-Step Guide
Now, let's meticulously trace the events of synaptic transmission in their chronological order:
1. Action Potential Arrival at the Presynaptic Terminal:
The process begins when an action potential – a rapid electrical signal – travels down the axon of the presynaptic neuron and reaches the presynaptic terminal (also known as the axon terminal or synaptic bouton). This action potential is an all-or-none event; its amplitude remains constant throughout its propagation.
2. Depolarization and Calcium Influx:
The arrival of the action potential triggers depolarization of the presynaptic terminal. This depolarization opens voltage-gated calcium (Ca²⁺) channels located in the presynaptic membrane. The electrochemical gradient drives a rapid influx of Ca²⁺ ions into the presynaptic terminal. The concentration of Ca²⁺ ions within the terminal increases dramatically. This increase is crucial for the next step.
3. Vesicle Fusion and Neurotransmitter Release (Exocytosis):
The rise in intracellular Ca²⁺ concentration triggers a cascade of events leading to the fusion of synaptic vesicles with the presynaptic membrane. This fusion process, known as exocytosis, releases the neurotransmitters stored within the vesicles into the synaptic cleft. The number of vesicles that fuse and the amount of neurotransmitter released are directly proportional to the amount of Ca²⁺ influx. This is a highly regulated process ensuring the precise control of neurotransmitter release.
4. Diffusion Across the Synaptic Cleft:
Once released into the synaptic cleft, the neurotransmitters diffuse across the narrow gap to reach the postsynaptic membrane. This diffusion is a passive process driven by the concentration gradient, with neurotransmitters moving from an area of high concentration (the cleft) to an area of lower concentration (the postsynaptic membrane).
5. Neurotransmitter Binding to Postsynaptic Receptors:
Neurotransmitters bind to specific receptor proteins located on the postsynaptic membrane. These receptors are highly selective; each receptor type binds only to specific neurotransmitters. This binding event is highly specific and is often described as a “lock and key” mechanism, analogous to enzyme-substrate binding.
6. Postsynaptic Potential Generation:
The binding of neurotransmitters to their receptors causes a change in the permeability of the postsynaptic membrane to ions. This change leads to the generation of a postsynaptic potential (PSP). There are two main types of PSPs:
-
Excitatory Postsynaptic Potentials (EPSPs): EPSPs are depolarizations of the postsynaptic membrane, making the postsynaptic neuron more likely to fire an action potential. They are typically caused by the opening of sodium (Na⁺) or calcium (Ca²⁺) channels.
-
Inhibitory Postsynaptic Potentials (IPSPs): IPSPs are hyperpolarizations of the postsynaptic membrane, making the postsynaptic neuron less likely to fire an action potential. They are typically caused by the opening of potassium (K⁺) or chloride (Cl⁻) channels.
7. Signal Termination:
The effects of the neurotransmitter binding must be terminated to prevent continuous stimulation or inhibition of the postsynaptic neuron. This termination occurs through several mechanisms:
-
Reuptake: Neurotransmitters are actively transported back into the presynaptic terminal by transporter proteins. This process is energy-dependent and allows for the recycling of neurotransmitters.
-
Enzymatic Degradation: Enzymes in the synaptic cleft break down neurotransmitters into inactive metabolites. For example, acetylcholinesterase breaks down acetylcholine.
-
Diffusion: Some neurotransmitters simply diffuse away from the synaptic cleft, reducing their concentration and thus their effects.
The Summation of Postsynaptic Potentials: A Balancing Act
A single EPSP or IPSP is rarely sufficient to trigger an action potential in the postsynaptic neuron. Instead, the postsynaptic neuron integrates the effects of multiple PSPs through a process called summation. There are two main types of summation:
-
Temporal Summation: Multiple PSPs from the same presynaptic neuron occurring in rapid succession. If the frequency of EPSPs is high enough, they can summate to reach the threshold for action potential generation.
-
Spatial Summation: PSPs from multiple presynaptic neurons arriving at the same time. The combined effect of multiple EPSPs from different sources can reach the threshold, even if individual EPSPs are subthreshold.
The postsynaptic neuron acts as a sophisticated integrator, weighing the combined effects of EPSPs and IPSPs to determine whether or not to fire an action potential. This intricate balance allows for complex information processing in the nervous system.
Modulation of Synaptic Transmission: Fine-Tuning the Signal
Synaptic transmission is not a static process; it is highly dynamic and subject to various modulatory influences:
-
Presynaptic Inhibition/Facilitation: The release of neurotransmitters from the presynaptic terminal can be modulated by other neurons that synapse onto the presynaptic terminal itself. This allows for precise control of neurotransmitter release.
-
Long-Term Potentiation (LTP): LTP is a persistent strengthening of synapses based on recent patterns of activity. It is thought to be a crucial mechanism underlying learning and memory.
-
Long-Term Depression (LTD): LTD is a persistent weakening of synapses. It plays a crucial role in synaptic plasticity and refining neural circuits.
-
Drug Interactions: Many drugs act by affecting synaptic transmission. For example, antidepressants often target neurotransmitter reuptake mechanisms, while many anesthetics act by blocking ion channels.
Clinical Significance: When Synaptic Transmission Goes Wrong
Disruptions in synaptic transmission underlie many neurological and psychiatric disorders. Examples include:
-
Alzheimer's disease: Characterized by impaired cholinergic neurotransmission.
-
Parkinson's disease: Involves a deficiency of dopamine in the brain.
-
Depression: Associated with imbalances in several neurotransmitter systems, including serotonin and dopamine.
-
Epilepsy: Often involves hyperexcitability of neurons, leading to uncontrolled firing of action potentials.
Conclusion: A Complex and Dynamic Process
The events of synaptic transmission, while seemingly simple when presented step-by-step, constitute a remarkably complex and precisely regulated process. The intricate interplay between different molecular components, the integration of multiple signals, and the dynamic modulation of synaptic strength ensure the efficient and flexible communication necessary for the functioning of the nervous system. A thorough understanding of these processes is essential for advancing our knowledge of brain function, disease mechanisms, and the development of effective therapeutic strategies. Further research continues to uncover new nuances and subtleties of this fundamental process, constantly refining our understanding of this cornerstone of neuroscience.
Latest Posts
Latest Posts
-
Give The Structure Of The Organic Product Expected When Ch2i2
Apr 11, 2025
-
Theoretical Basis For Nursing 6th Edition Ebook
Apr 11, 2025
-
A Car Is Traveling Up A Hill That Is Inclined
Apr 11, 2025
-
A Voltage Tester Gives Only Approximate Voltage Measurements
Apr 11, 2025
-
Draw The Lewis Structure For The Polyatomic Trisulfide Anion
Apr 11, 2025
Related Post
Thank you for visiting our website which covers about Place The Events Of Synaptic Transmission In Order . We hope the information provided has been useful to you. Feel free to contact us if you have any questions or need further assistance. See you next time and don't miss to bookmark.