Photosystem I And Photosystem Ii Are Respectively Part Of
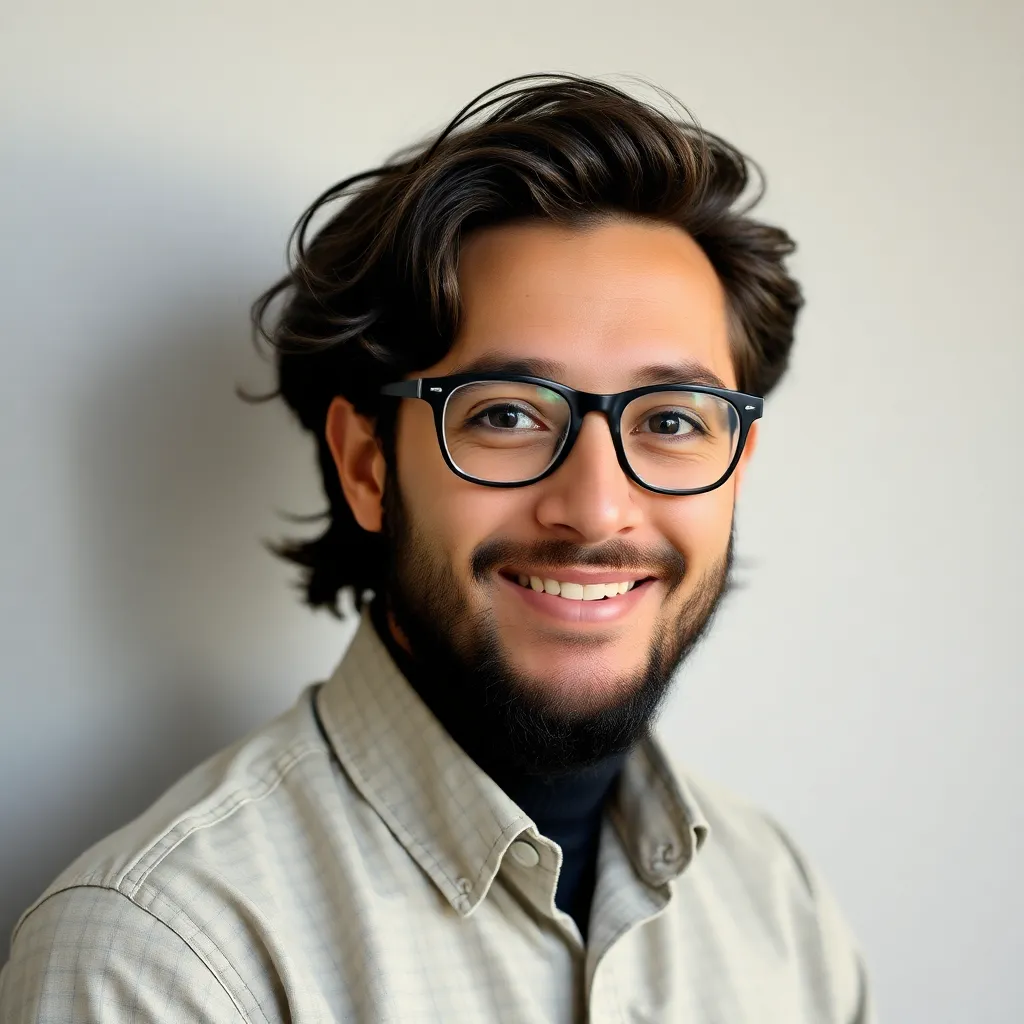
Holbox
Mar 29, 2025 · 6 min read
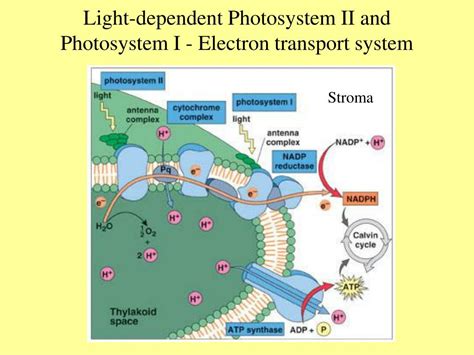
Table of Contents
- Photosystem I And Photosystem Ii Are Respectively Part Of
- Table of Contents
- Photosystem I and Photosystem II: Respectively Part of the Light-Dependent Reactions in Photosynthesis
- Photosystem II: The Initial Light Harvester
- 1. Light-Harvesting Complexes (LHCs): Expanding the Absorption Spectrum
- 2. The Reaction Center: The Heart of PSII
- 3. Water Splitting: The Source of Electrons
- 4. The Electron Transport Chain: Generating a Proton Gradient
- Photosystem I: Continuing the Electron Flow and NADPH Production
- 1. Light Absorption and Electron Transfer in PSI
- 2. Ferredoxin and NADP+ Reductase: NADPH Synthesis
- 3. Cyclic Electron Flow: Generating Additional ATP
- The Interplay Between Photosystem I and Photosystem II: A Coordinated Effort
- Chemiosmosis: Harnessing the Proton Gradient
- The Importance of Accessory Pigments: Expanding the Range of Light Absorption
- Regulation of Photosystem Activity: Optimizing Photosynthesis
- Photosystem I and Photosystem II: Evolutionary Significance and Diversity
- Beyond the Basics: Future Research and Applications
- Conclusion: The Heart of Photosynthesis
- Latest Posts
- Latest Posts
- Related Post
Photosystem I and Photosystem II: Respectively Part of the Light-Dependent Reactions in Photosynthesis
Photosynthesis, the remarkable process by which plants and other organisms convert light energy into chemical energy, is underpinned by a series of intricate reactions. Central to this process are two vital protein complexes: Photosystem I (PSI) and Photosystem II (PSII). These photosystems, embedded within the thylakoid membranes of chloroplasts, work in concert to drive the light-dependent reactions, ultimately fueling the synthesis of glucose. Understanding their respective roles and interconnectedness is crucial to grasping the intricacies of photosynthesis.
Photosystem II: The Initial Light Harvester
Photosystem II, often abbreviated as PSII, initiates the light-dependent reactions by absorbing light energy. This absorption is not a singular event but rather a carefully orchestrated process involving several key components:
1. Light-Harvesting Complexes (LHCs): Expanding the Absorption Spectrum
PSII doesn't work in isolation. Surrounding it are light-harvesting complexes (LHCs), also known as antenna complexes. These LHCs consist of numerous chlorophyll a and chlorophyll b molecules, along with carotenoids. The arrangement of these pigments allows PSII to capture a broader spectrum of light than it could alone. Different pigments absorb different wavelengths of light, effectively widening the range of usable solar energy. The absorbed energy is then funneled towards the reaction center of PSII.
2. The Reaction Center: The Heart of PSII
At the core of PSII lies the reaction center, a chlorophyll a dimer known as P680. This dimer is special because it's capable of transferring an excited electron to a primary electron acceptor, pheophytin. The absorption of light energy by P680 boosts an electron to a higher energy level, initiating the electron transport chain. This electron transfer is crucial for the next stage of photosynthesis.
3. Water Splitting: The Source of Electrons
The departure of an electron from P680 leaves a "hole" that needs to be filled. PSII achieves this remarkable feat through water splitting, a process catalyzed by the oxygen-evolving complex (OEC). The OEC, a manganese-containing cluster, extracts electrons from water molecules, splitting them into oxygen (O2), protons (H+), and electrons. This process is not only essential for replenishing the electrons lost by P680 but also releases the oxygen we breathe.
4. The Electron Transport Chain: Generating a Proton Gradient
The electron ejected from P680 doesn't travel directly to PSI. Instead, it embarks on a journey through the electron transport chain. This chain consists of several electron carriers, including plastoquinone (PQ), cytochrome b6f complex, and plastocyanin (PC). As the electron moves along this chain, energy is released, used to pump protons (H+) from the stroma into the thylakoid lumen. This creates a proton gradient across the thylakoid membrane, a crucial driving force for ATP synthesis.
Photosystem I: Continuing the Electron Flow and NADPH Production
After its journey through the electron transport chain, the electron ultimately reaches Photosystem I (PSI). PSI, similar to PSII, also contains a reaction center and light-harvesting complexes.
1. Light Absorption and Electron Transfer in PSI
The reaction center of PSI, containing a chlorophyll a dimer called P700, absorbs light energy. This excitation boosts an electron to a higher energy level, enabling its transfer to a primary electron acceptor, usually a chlorophyll molecule or phylloquinone.
2. Ferredoxin and NADP+ Reductase: NADPH Synthesis
Unlike PSII, the electron flow in PSI doesn't lead directly to proton pumping. Instead, the electron travels through a series of electron carriers, including ferredoxin (Fd), ultimately reaching NADP+ reductase. This enzyme catalyzes the reduction of NADP+ to NADPH, a crucial electron carrier vital for the light-independent reactions (Calvin cycle).
3. Cyclic Electron Flow: Generating Additional ATP
While the linear electron flow described above is essential, PSI can also participate in cyclic electron flow. In this process, the electron, after being excited by P700, cycles back to the cytochrome b6f complex, contributing to further proton pumping and ATP generation. This mechanism is particularly important when the demand for ATP exceeds the need for NADPH.
The Interplay Between Photosystem I and Photosystem II: A Coordinated Effort
Photosystem I and Photosystem II are not independent entities but rather integral components of a tightly regulated system. Their cooperation is crucial for the efficient conversion of light energy into chemical energy. The electrons extracted from water by PSII are ultimately used to reduce NADP+ to NADPH in PSI. This electron flow is coupled with proton pumping, establishing a proton gradient that drives ATP synthesis via chemiosmosis.
Chemiosmosis: Harnessing the Proton Gradient
The proton gradient generated by both PSII and (potentially through cyclic electron flow) PSI is harnessed by ATP synthase, an enzyme embedded in the thylakoid membrane. ATP synthase utilizes the energy stored in the proton gradient to synthesize ATP, the cellular energy currency. This process, known as chemiosmosis, is essential for powering the energy-demanding reactions of the Calvin cycle.
The Importance of Accessory Pigments: Expanding the Range of Light Absorption
Both PSI and PSII benefit from accessory pigments. Chlorophyll b and carotenoids play a critical role in expanding the range of wavelengths absorbed by the photosystems. These pigments absorb light energy and then transfer it to chlorophyll a molecules in the reaction centers, enhancing the overall efficiency of light harvesting. Carotenoids, in addition to their role in light harvesting, also protect the photosystems from photodamage caused by excessive light energy.
Regulation of Photosystem Activity: Optimizing Photosynthesis
The activity of PSI and PSII is not static; it's precisely regulated to optimize photosynthesis under varying light conditions. Several factors influence this regulation, including light intensity, the availability of water, and the concentrations of ATP and NADPH. This intricate regulation ensures that the process of photosynthesis is efficiently adjusted to environmental cues.
Photosystem I and Photosystem II: Evolutionary Significance and Diversity
The evolution of PSI and PSII represents a pivotal step in the history of life on Earth. These photosystems enabled the development of oxygenic photosynthesis, dramatically reshaping Earth's atmosphere and paving the way for the evolution of complex life forms. Moreover, the structure and function of these photosystems exhibit considerable diversity across different plant species and organisms, reflecting adaptations to various environmental conditions.
Beyond the Basics: Future Research and Applications
Research on photosystems continues to unveil new details about their structure, function, and regulation. Understanding the intricate mechanisms of photosynthesis holds immense potential for various applications, including:
- Bioenergy: Harnessing the power of photosynthesis to generate renewable biofuels.
- Agriculture: Improving crop yields by enhancing photosynthetic efficiency.
- Environmental remediation: Utilizing photosynthetic organisms to remove pollutants from the environment.
Conclusion: The Heart of Photosynthesis
Photosystem I and Photosystem II, working in tandem, form the core of the light-dependent reactions in photosynthesis. Their intricate interplay, involving light absorption, electron transfer, proton pumping, and ATP and NADPH synthesis, represents a remarkable feat of biological engineering. Continued research into these photosystems promises to unlock further insights into the fundamental processes of life and inspire innovative solutions to global challenges. The ongoing exploration of these vital components of photosynthesis emphasizes their critical role in sustaining life on Earth and their potential for future technological advancements.
Latest Posts
Latest Posts
-
Humans Carry A Variety Of Non Functional Genetic Sequences Called
Apr 02, 2025
-
Michael Is An Art Elective Programme Student
Apr 02, 2025
-
A Large Negative Gdp Gap Implies
Apr 02, 2025
-
Which Of The Techniques Are Examples Of Biotechnology
Apr 02, 2025
-
The Scores Of A Recent Test Taken By 1200
Apr 02, 2025
Related Post
Thank you for visiting our website which covers about Photosystem I And Photosystem Ii Are Respectively Part Of . We hope the information provided has been useful to you. Feel free to contact us if you have any questions or need further assistance. See you next time and don't miss to bookmark.