Pair Up The Nucleotide Bases With Their Complementary Partners.
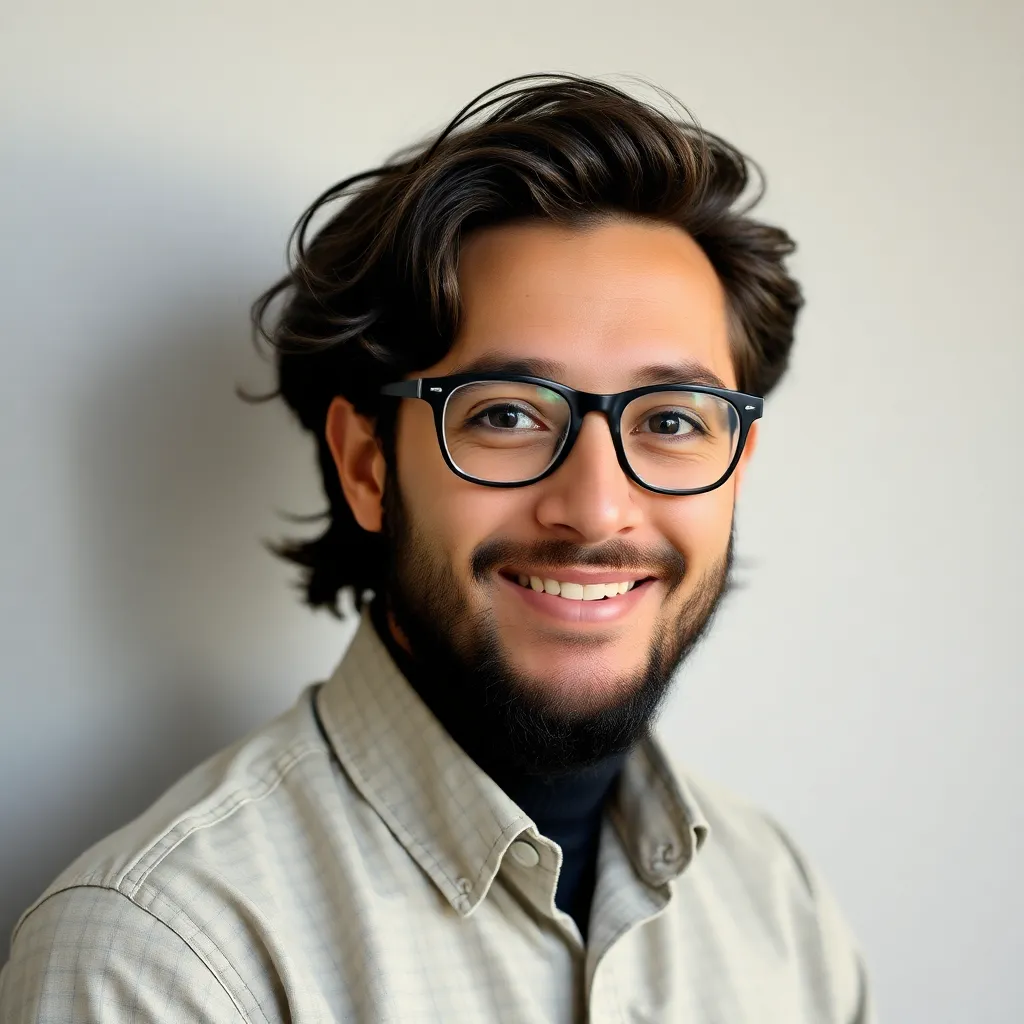
Holbox
Apr 08, 2025 · 6 min read

Table of Contents
- Pair Up The Nucleotide Bases With Their Complementary Partners.
- Table of Contents
- Pair Up the Nucleotide Bases with Their Complementary Partners: A Deep Dive into DNA and RNA
- The Four Main Nucleotide Bases: A Closer Look
- Adenine (A):
- Guanine (G):
- Cytosine (C):
- Thymine (T) and Uracil (U):
- The Fundamentals of Base Pairing: Chargaff's Rules and Watson-Crick Pairing
- Hydrogen Bonding: The Glue of Base Pairing
- Base Pairing in DNA Replication: Ensuring Faithful Copying
- Base Pairing in Transcription: From DNA to RNA
- Base Pairing in Translation: From RNA to Protein
- Beyond the Standard Base Pairs: Non-Watson-Crick Base Pairs and Wobble Pairing
- The Significance of Base Pairing in Medicine and Biotechnology
- Diagnostics:
- Gene Therapy:
- Pharmaceuticals:
- Conclusion: The Enduring Importance of Base Pairing
- Latest Posts
- Latest Posts
- Related Post
Pair Up the Nucleotide Bases with Their Complementary Partners: A Deep Dive into DNA and RNA
The elegance of life lies, in part, within the intricate dance of its building blocks. At the heart of this dance are the nucleotide bases, the fundamental units that form the backbone of DNA and RNA, the genetic blueprints of all living organisms. Understanding how these bases pair up—a process critical to DNA replication, transcription, and translation—is essential to understanding life itself. This comprehensive article delves into the fascinating world of nucleotide base pairing, exploring their structures, interactions, and significance in various biological processes.
The Four Main Nucleotide Bases: A Closer Look
Before delving into base pairing, let's familiarize ourselves with the key players: adenine (A), guanine (G), cytosine (C), and thymine (T). In RNA, uracil (U) replaces thymine. Each base possesses a unique chemical structure that dictates its complementary pairing.
Adenine (A):
Adenine is a purine base, meaning it's a double-ringed structure composed of a six-membered ring fused to a five-membered ring. This structure is crucial for its specific interaction with its complementary base.
Guanine (G):
Also a purine, guanine shares a similar double-ringed structure with adenine, but with key differences in its functional groups that dictate its pairing specificity.
Cytosine (C):
Cytosine is a pyrimidine base, characterized by a single six-membered ring structure. Its unique arrangement of functional groups allows for highly specific pairing with its complementary partner.
Thymine (T) and Uracil (U):
Both thymine and uracil are pyrimidine bases, differing only by a single methyl group. Thymine is found exclusively in DNA, while uracil substitutes for thymine in RNA. This subtle structural difference has significant implications for the stability and function of DNA and RNA.
The Fundamentals of Base Pairing: Chargaff's Rules and Watson-Crick Pairing
The foundation of our understanding of base pairing rests on Chargaff's rules and the subsequent Watson-Crick model. Erwin Chargaff, through meticulous experimentation, observed that in DNA, the amount of adenine (A) always equals the amount of thymine (T), and the amount of guanine (G) always equals the amount of cytosine (C). This observation, known as Chargaff's rules, hinted at a fundamental pairing mechanism.
The Watson-Crick model, proposed by James Watson and Francis Crick, elegantly explained Chargaff's rules. They showed that A pairs with T, and G pairs with C, through the formation of hydrogen bonds.
Hydrogen Bonding: The Glue of Base Pairing
Hydrogen bonds are weak, non-covalent bonds that form between a hydrogen atom and an electronegative atom (like oxygen or nitrogen). These bonds are individually weak, but collectively, the multiple hydrogen bonds formed between complementary base pairs provide significant stability to the DNA double helix.
-
Adenine-Thymine (A-T) Pairing: Two hydrogen bonds form between A and T. A's amino group forms a hydrogen bond with T's carbonyl group, and A's other nitrogen forms a hydrogen bond with T's other carbonyl group.
-
Guanine-Cytosine (G-C) Pairing: Three hydrogen bonds form between G and C. The increased number of hydrogen bonds makes the G-C base pair stronger than the A-T base pair. This difference in bond strength influences DNA stability and melting temperature.
Base Pairing in DNA Replication: Ensuring Faithful Copying
The precise pairing of nucleotide bases is paramount in DNA replication, the process of creating an exact copy of the DNA molecule. During replication, the DNA double helix unwinds, and each strand serves as a template for the synthesis of a new complementary strand. DNA polymerase, an enzyme that plays a critical role in the process, ensures that the correct nucleotides are added to the new strand based on the base-pairing rules.
The fidelity of DNA replication is crucial for maintaining genetic stability. Mistakes during replication, though rare, can lead to mutations, which may have profound effects on the organism. DNA repair mechanisms exist to correct many of these errors, minimizing the potential consequences of replication mistakes.
Base Pairing in Transcription: From DNA to RNA
Transcription is the process of synthesizing RNA from a DNA template. Similar to DNA replication, base pairing plays a critical role in this process. The enzyme RNA polymerase unwinds the DNA double helix and uses one strand as a template to synthesize a complementary RNA molecule.
However, there's a key difference: uracil (U) replaces thymine (T) in RNA. Therefore, adenine (A) in the DNA template pairs with uracil (U) in the RNA transcript, while guanine (G) still pairs with cytosine (C). This process ensures that the genetic information encoded in DNA is accurately transcribed into RNA.
Base Pairing in Translation: From RNA to Protein
Translation is the process of converting the genetic information encoded in mRNA (messenger RNA) into a protein sequence. This process occurs in ribosomes, cellular structures that read the mRNA sequence and assemble the corresponding amino acid chain.
While base pairing is not directly involved in the formation of peptide bonds between amino acids, it's essential for the accurate decoding of the mRNA message. Transfer RNA (tRNA) molecules, each carrying a specific amino acid, recognize and bind to complementary codons (three-nucleotide sequences) on the mRNA through base pairing. This ensures that the correct amino acids are incorporated into the growing polypeptide chain, leading to the synthesis of a functional protein.
Beyond the Standard Base Pairs: Non-Watson-Crick Base Pairs and Wobble Pairing
While the Watson-Crick base pairs (A-T and G-C) are the dominant pairing interactions, other non-Watson-Crick base pairs can occur, particularly in RNA structures. These non-canonical base pairs often contribute to the complex three-dimensional folding of RNA molecules, which is essential for their function.
Wobble pairing is a type of non-Watson-Crick base pairing that occurs specifically in the third position of a codon-anticodon interaction during translation. This flexibility in base pairing allows a single tRNA molecule to recognize multiple codons, thereby increasing the efficiency of the translation process.
The Significance of Base Pairing in Medicine and Biotechnology
The principles of base pairing are not merely academic curiosities; they form the cornerstone of many advancements in medicine and biotechnology.
Diagnostics:
Understanding base pairing allows for the development of diagnostic tools, such as PCR (polymerase chain reaction), which is used to amplify specific DNA sequences for disease detection.
Gene Therapy:
Gene therapy relies on the precise manipulation of DNA sequences, employing the principles of base pairing to introduce functional genes into cells to correct genetic defects.
Pharmaceuticals:
Many pharmaceuticals target specific DNA or RNA sequences through base pairing interactions, disrupting harmful processes or enhancing beneficial ones. Antisense technology, for example, utilizes short RNA molecules designed to bind to complementary mRNA sequences, thereby preventing protein synthesis.
Conclusion: The Enduring Importance of Base Pairing
The seemingly simple pairing of nucleotide bases – A with T (or U), and G with C – is a fundamental process that underpins the very essence of life. From DNA replication to protein synthesis, the precise and faithful pairing of these bases ensures the accurate transmission of genetic information, allowing for the development, growth, and reproduction of all living organisms. A deep understanding of base pairing is crucial not only for comprehending the intricacies of life itself but also for developing cutting-edge advancements in medicine and biotechnology, shaping the future of healthcare and scientific discovery. The ongoing research into the nuances of base pairing and its implications continues to unveil new insights into the complexity and elegance of the biological world.
Latest Posts
Latest Posts
-
Without Using Parentheses Enter A Formula
Apr 15, 2025
-
The Norton Field Guide To Writing With Readings 6th Edition
Apr 15, 2025
-
In An Informative Speech The Speaker Acts As
Apr 15, 2025
-
A Linear Programming Problem Can Have Multiple Optimal Solutions
Apr 15, 2025
-
Economic Power Is Diffused Throughout An Economy When
Apr 15, 2025
Related Post
Thank you for visiting our website which covers about Pair Up The Nucleotide Bases With Their Complementary Partners. . We hope the information provided has been useful to you. Feel free to contact us if you have any questions or need further assistance. See you next time and don't miss to bookmark.