Order The Steps Of Atp Synthesis By Atp Synthase
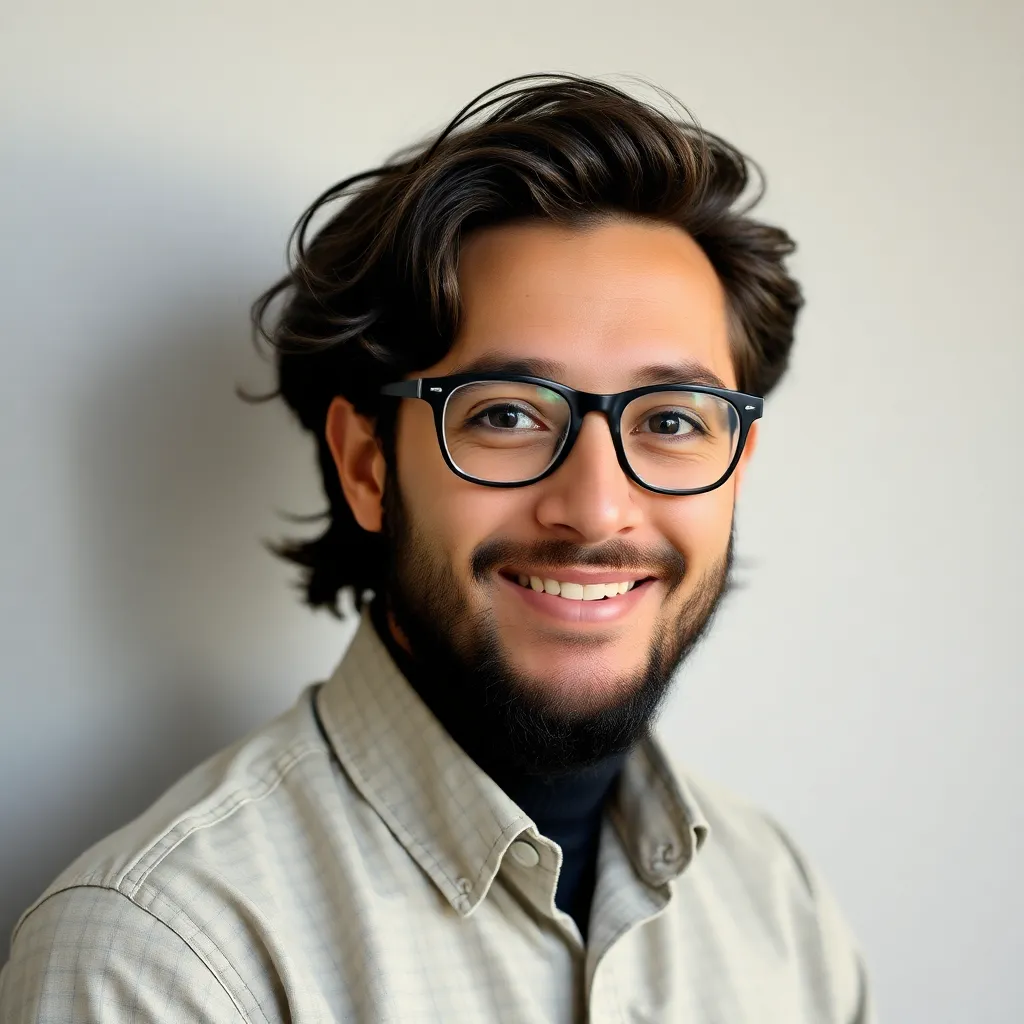
Holbox
May 12, 2025 · 6 min read
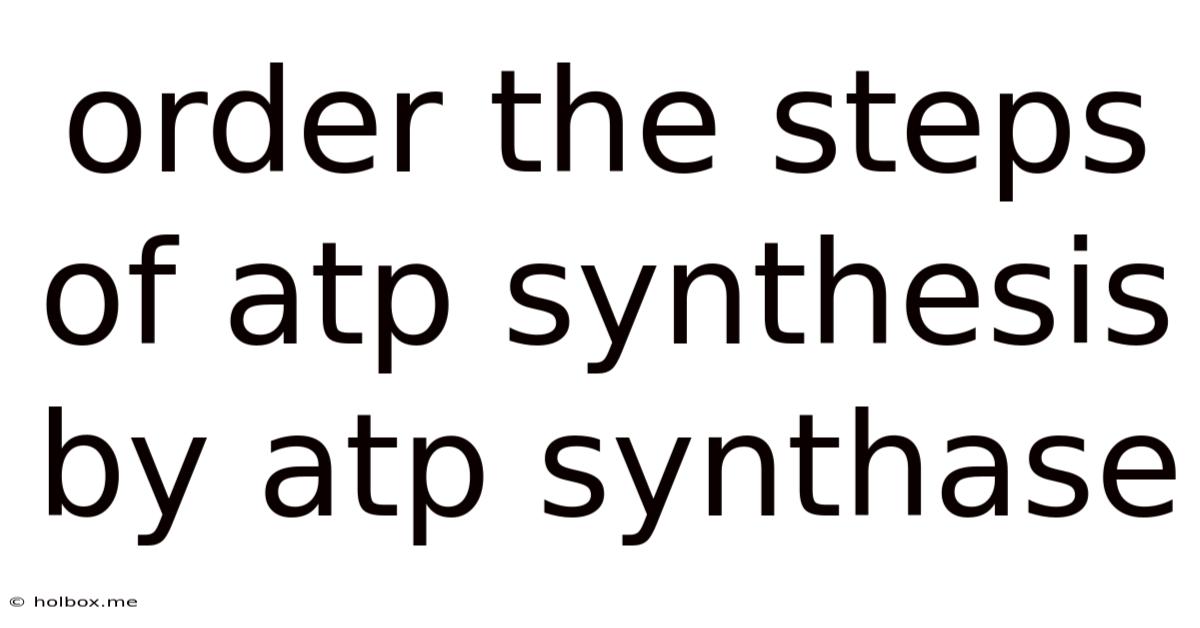
Table of Contents
- Order The Steps Of Atp Synthesis By Atp Synthase
- Table of Contents
- Ordering the Steps of ATP Synthesis by ATP Synthase: A Comprehensive Guide
- The Structure of ATP Synthase: A Foundation for Understanding Function
- 1. F<sub>0</sub> Subunit: The Proton Channel
- 2. F<sub>1</sub> Subunit: The Catalytic Core
- The Rotary Catalysis Mechanism: A Step-by-Step Guide to ATP Synthesis
- Step 1: Proton Binding and c-Ring Rotation
- Step 2: Rotation of the γ Subunit and Conformational Changes in β Subunits
- Step 3: ATP Synthesis in the Tight (T) State
- Step 4: ATP Release and Cycle Continuation
- The Role of the Proton Motive Force (PMF)
- Regulation of ATP Synthase Activity
- ATP Synthase in Different Organisms
- Further Research and Future Directions
- Conclusion
- Latest Posts
- Latest Posts
- Related Post
Ordering the Steps of ATP Synthesis by ATP Synthase: A Comprehensive Guide
ATP synthase, a remarkable molecular machine, is responsible for the majority of ATP (adenosine triphosphate) production in living organisms. This enzyme, found in the inner mitochondrial membrane of eukaryotes and the plasma membrane of prokaryotes, harnesses the energy stored in a proton gradient to synthesize ATP, the cell's primary energy currency. Understanding the precise steps involved in ATP synthesis by ATP synthase is crucial to grasping cellular energy metabolism. This article provides a detailed, step-by-step breakdown of this process, incorporating relevant keywords for improved SEO and readability.
The Structure of ATP Synthase: A Foundation for Understanding Function
Before delving into the mechanism of ATP synthesis, it's vital to understand the structure of ATP synthase. This enzyme is a complex molecular machine consisting of two main components:
1. F<sub>0</sub> Subunit: The Proton Channel
The F<sub>0</sub> subunit is embedded within the membrane and acts as a proton channel. It's composed of multiple subunits (a, b, and c), with the c-subunit forming a ring that rotates upon proton translocation. This rotation is the key to driving ATP synthesis. The 'a' subunit acts as a stator, anchoring the F<sub>0</sub> subunit to the membrane and providing a pathway for protons to reach the c-ring. The 'b' subunits act as a bridge between F<sub>0</sub> and F<sub>1</sub>. The exact number of c-subunits varies depending on the organism, but it's typically around 10-14.
2. F<sub>1</sub> Subunit: The Catalytic Core
The F<sub>1</sub> subunit protrudes into the mitochondrial matrix (or cytoplasm in prokaryotes) and houses the catalytic sites for ATP synthesis. It's composed of five different subunits (α<sub>3</sub>, β<sub>3</sub>, γ, δ, and ε), arranged in a unique structure. Three of the β subunits are catalytic, each capable of binding and converting ADP and inorganic phosphate (Pi) into ATP. The α subunits play a regulatory role, and the γ, δ, and ε subunits form a central stalk that connects to the F<sub>0</sub> subunit, transmitting the rotational force. This central stalk is crucial in coordinating the conformational changes required for ATP synthesis.
The Rotary Catalysis Mechanism: A Step-by-Step Guide to ATP Synthesis
The mechanism of ATP synthesis by ATP synthase is elegantly described as rotary catalysis. The flow of protons through the F<sub>0</sub> subunit drives the rotation of the c-ring, which in turn rotates the γ subunit within the F<sub>1</sub> subunit. This rotation induces conformational changes in the three β subunits, cycling them through different states, leading to ATP synthesis. Here's a step-by-step breakdown:
Step 1: Proton Binding and c-Ring Rotation
Protons (H<sup>+</sup>) from the high-proton-concentration side of the membrane bind to the c-subunits of the F<sub>0</sub> subunit. This binding triggers a conformational change in the c-subunit, causing the entire c-ring to rotate. Each proton binding event contributes to the rotation, akin to stepping along a staircase.
Step 2: Rotation of the γ Subunit and Conformational Changes in β Subunits
The rotation of the c-ring is mechanically coupled to the rotation of the γ subunit within the F<sub>1</sub> subunit. This rotation acts as a central driver, altering the conformation of the three catalytic β subunits. These conformational changes are crucial to ATP synthesis, transitioning each β subunit sequentially through three different states:
-
Open (O) state: The β subunit has low affinity for both ADP and Pi, so these molecules can readily bind or dissociate.
-
Loose (L) state: The β subunit binds ADP and Pi with moderate affinity, preparing them for catalysis.
-
Tight (T) state: The β subunit binds ADP and Pi tightly, facilitating the formation of ATP.
Step 3: ATP Synthesis in the Tight (T) State
As the γ subunit rotates, it forces one β subunit into the tight (T) state. In this state, the bound ADP and Pi are brought into close proximity, allowing them to react and form ATP. The energy for this reaction is derived from the proton gradient, indirectly via the rotational movement.
Step 4: ATP Release and Cycle Continuation
The continued rotation of the γ subunit shifts the β subunit from the tight (T) state to the open (O) state, releasing the newly synthesized ATP. The cycle then repeats, with other β subunits moving through the same O, L, and T states, resulting in continuous ATP synthesis.
The Role of the Proton Motive Force (PMF)
The entire process is driven by the proton motive force (PMF), which is composed of two components:
-
Proton concentration gradient (ΔpH): The difference in proton concentration across the membrane.
-
Membrane potential (ΔΨ): The electrical potential difference across the membrane, caused by the uneven distribution of charge.
The PMF represents the stored energy from electron transport, which is used to pump protons across the membrane, creating the gradient that drives ATP synthesis. The precise contribution of each component (ΔpH and ΔΨ) varies depending on the organism and physiological conditions.
Regulation of ATP Synthase Activity
ATP synthase activity is tightly regulated to meet cellular energy demands. Several factors influence its activity:
-
ATP/ADP ratio: A high ATP/ADP ratio inhibits ATP synthase activity, preventing the wasteful synthesis of ATP when energy levels are already high.
-
Inhibitors: Certain molecules, such as oligomycin, can specifically inhibit ATP synthase activity by binding to the F<sub>0</sub> subunit and blocking proton flow.
-
Activators: Conversely, some molecules can activate ATP synthase, such as certain divalent cations.
ATP Synthase in Different Organisms
While the fundamental mechanism of ATP synthase is conserved across various organisms, there are some variations:
-
Bacterial ATP Synthase: Bacterial ATP synthases generally have a smaller c-ring than mitochondrial ATP synthases.
-
Archaeal ATP Synthase: Archaeal ATP synthases exhibit structural differences in the F<sub>0</sub> subunit.
-
Chloroplast ATP Synthase: Chloroplast ATP synthases, involved in photosynthesis, operate similarly but utilize a different proton gradient generated across the thylakoid membrane.
Further Research and Future Directions
ATP synthase remains a fascinating area of research. Scientists continue to investigate aspects such as:
-
Detailed structural dynamics: Employing advanced techniques to understand the precise conformational changes during ATP synthesis.
-
Regulation mechanisms: Identifying and characterizing additional regulatory mechanisms that fine-tune ATP synthase activity.
-
Therapeutic applications: Exploring the potential of targeting ATP synthase for drug development, particularly in the context of diseases related to mitochondrial dysfunction.
Conclusion
ATP synthesis by ATP synthase is a remarkable example of biological efficiency, converting the energy stored in a proton gradient into the cell's primary energy currency. Understanding the ordered steps involved in this process, from proton translocation to ATP release, is essential for appreciating cellular energy metabolism. The intricate structure and dynamic function of ATP synthase continue to inspire scientific inquiry, promising further advancements in our understanding of this fundamental biological process. Further research promises to uncover even more details about this incredible molecular machine and its crucial role in life.
Latest Posts
Latest Posts
-
What Is 23 Kg In Stones
May 20, 2025
-
How Many Weeks Is 39 Days
May 20, 2025
-
What Is 2 Litres In Pints
May 20, 2025
-
How Many Teaspoons Is 15 Ml
May 20, 2025
-
How Many Weeks Are In 52 Days
May 20, 2025
Related Post
Thank you for visiting our website which covers about Order The Steps Of Atp Synthesis By Atp Synthase . We hope the information provided has been useful to you. Feel free to contact us if you have any questions or need further assistance. See you next time and don't miss to bookmark.