Nucleic Acid Hybridization Is Based On The Fact That
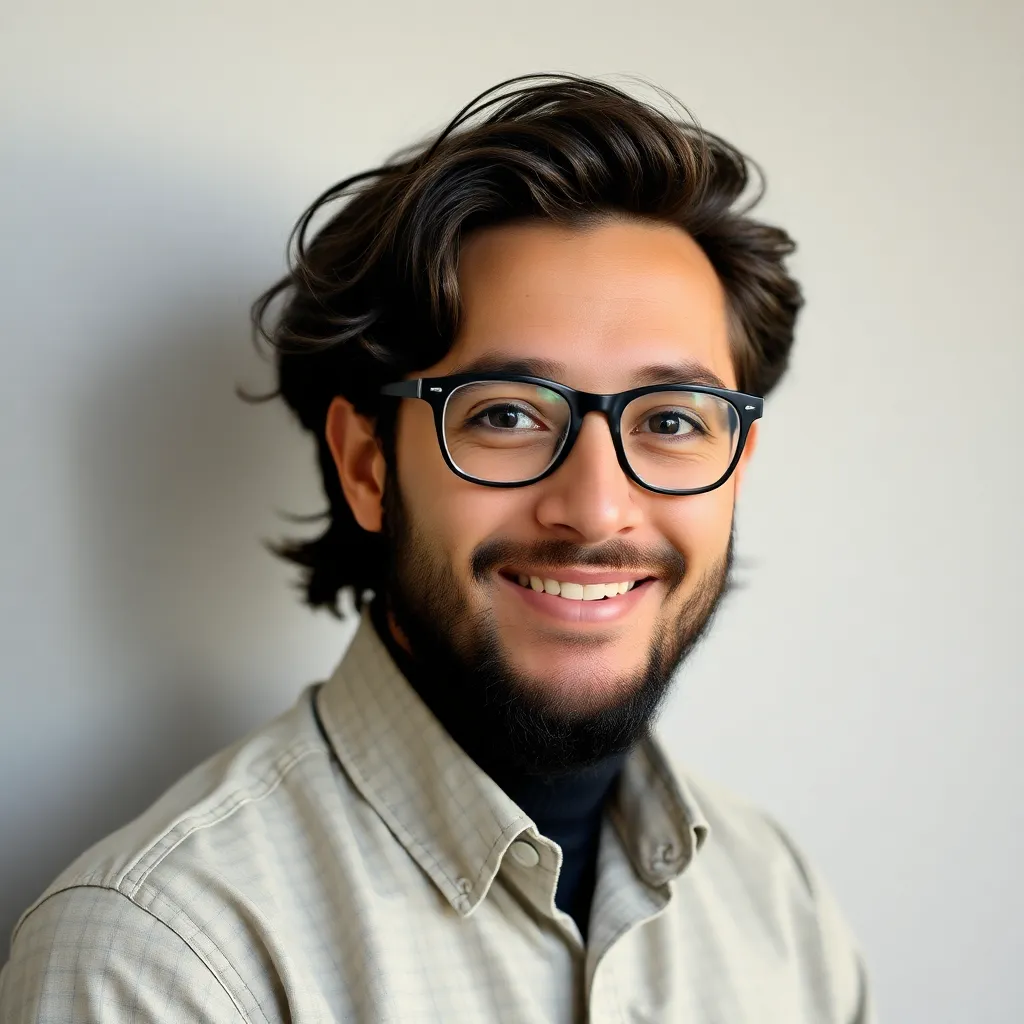
Holbox
Apr 15, 2025 · 6 min read
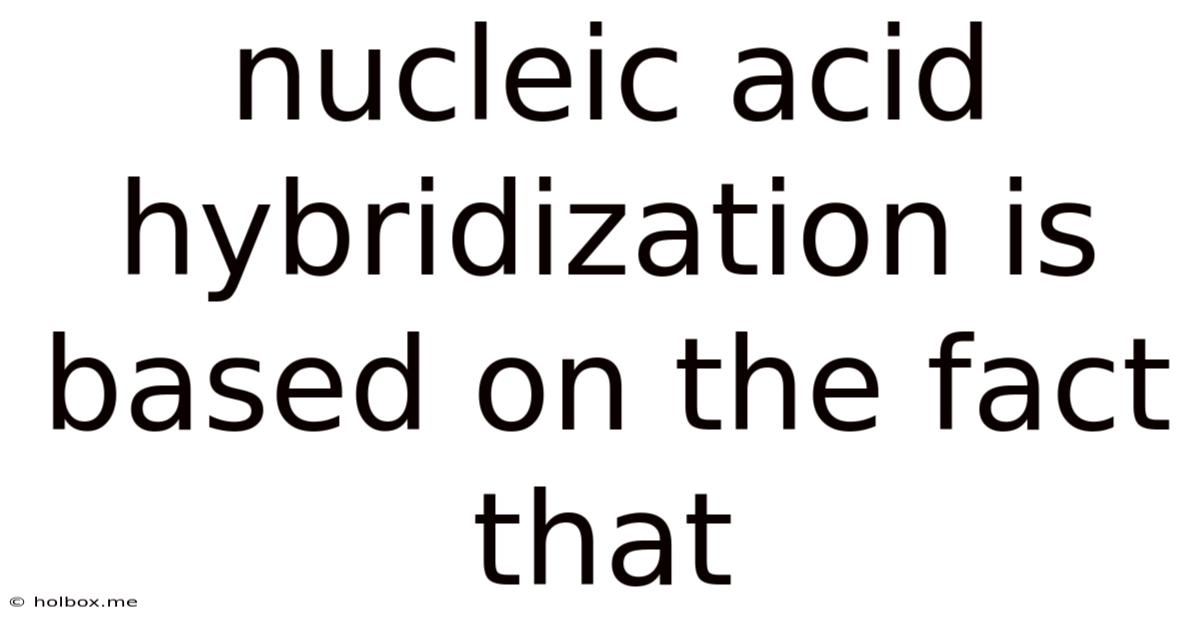
Table of Contents
- Nucleic Acid Hybridization Is Based On The Fact That
- Table of Contents
- Nucleic Acid Hybridization: A Foundation of Molecular Biology
- The Principle of Complementary Base Pairing
- Factors Affecting Hybridization
- Types of Nucleic Acid Hybridization Techniques
- 1. Southern Blotting
- 2. Northern Blotting
- 3. Western Blotting
- 4. In Situ Hybridization (ISH)
- 5. Microarrays
- 6. Polymerase Chain Reaction (PCR)
- 7. Quantitative PCR (qPCR)
- Applications of Nucleic Acid Hybridization
- 1. Medical Diagnostics
- 2. Forensic Science
- 3. Gene Expression Studies
- 4. Genetic Engineering
- 5. Phylogenetics
- 6. Genomics and Proteomics
- Future Directions
- Latest Posts
- Latest Posts
- Related Post
Nucleic Acid Hybridization: A Foundation of Molecular Biology
Nucleic acid hybridization is a fundamental technique in molecular biology based on the principle of complementary base pairing between nucleic acid strands. This powerful method exploits the specific interaction between adenine (A) and thymine (T) (or uracil (U) in RNA), and guanine (G) and cytosine (C), forming the foundation for a wide range of applications in research and diagnostics. Understanding this principle unlocks a world of possibilities in studying genes, diagnosing diseases, and advancing biotechnology.
The Principle of Complementary Base Pairing
At the heart of nucleic acid hybridization lies the ability of two single-stranded nucleic acid molecules – DNA or RNA – to form a stable double-stranded structure if they possess complementary sequences. This pairing is dictated by the specific hydrogen bonds that form between the bases: two hydrogen bonds between A and T (or U), and three hydrogen bonds between G and C. The strength of the resulting duplex depends on the length of the complementary sequences, the percentage of G-C base pairs (G-C pairs are stronger due to the extra hydrogen bond), and the conditions under which hybridization occurs (temperature, salt concentration).
Factors Affecting Hybridization
Several factors influence the efficiency and specificity of nucleic acid hybridization:
-
Temperature: Higher temperatures disrupt weaker hydrogen bonds, demanding a higher degree of complementarity for stable duplex formation. This is crucial for ensuring specific hybridization and minimizing non-specific binding. The optimal hybridization temperature, often referred to as the melting temperature (Tm), is determined experimentally and depends on the length and G-C content of the sequences.
-
Salt Concentration: The presence of salts in the hybridization buffer shields the negatively charged phosphate backbone of nucleic acids, reducing electrostatic repulsion and promoting duplex formation. Higher salt concentrations generally favor hybridization.
-
Sequence Length and Complementarity: Longer, perfectly complementary sequences form more stable hybrids than shorter or partially complementary sequences. Even a single mismatch can significantly reduce the stability of the duplex.
-
Formamide Concentration: Formamide is a commonly used denaturing agent that disrupts hydrogen bonding. Adding formamide lowers the Tm, allowing for hybridization at lower temperatures and increasing stringency.
Types of Nucleic Acid Hybridization Techniques
The principle of complementary base pairing is exploited in a variety of techniques, each adapted to specific applications:
1. Southern Blotting
Southern blotting utilizes DNA-DNA hybridization to detect specific DNA sequences within a complex mixture of DNA fragments. DNA is first digested with restriction enzymes, separated by gel electrophoresis, and then transferred to a membrane. A labeled probe (a single-stranded DNA fragment complementary to the target sequence) is then hybridized to the DNA on the membrane. The presence of the target sequence is revealed by detecting the labeled probe. This technique is widely used in gene mapping, genetic fingerprinting, and disease diagnosis.
2. Northern Blotting
Northern blotting, analogous to Southern blotting, involves RNA-DNA hybridization to detect specific RNA molecules. Total RNA is separated by gel electrophoresis, transferred to a membrane, and hybridized with a labeled DNA probe complementary to the target RNA sequence. This technique is employed to study gene expression levels and RNA processing.
3. Western Blotting
While not directly involving nucleic acid hybridization, Western blotting is closely related and worth mentioning. This technique detects specific proteins using antibodies. The proteins are separated by gel electrophoresis, transferred to a membrane, and then probed with antibodies that bind to the target protein. Although not a nucleic acid hybridization method, it shares a similar methodology of separation, transfer, and probe detection.
4. In Situ Hybridization (ISH)
ISH is a powerful technique that allows the localization of specific nucleic acid sequences within cells or tissues. A labeled probe is directly hybridized to the nucleic acids in place, revealing the spatial distribution of the target sequence. Different types of ISH exist, including fluorescence in situ hybridization (FISH), which uses fluorescently labeled probes, making it easily visualized under a fluorescence microscope. ISH is crucial in cytogenetics, cancer research, and developmental biology.
5. Microarrays
Microarrays employ a high-throughput approach to analyze the expression levels of thousands of genes simultaneously. Thousands of DNA probes, each representing a specific gene, are spotted onto a solid support (e.g., a glass slide). Labeled cDNA or cRNA, prepared from a sample, is hybridized to the microarray. The intensity of the signal at each spot reflects the abundance of the corresponding transcript in the sample. Microarrays are extensively used in gene expression profiling, disease diagnosis, and drug discovery.
6. Polymerase Chain Reaction (PCR)
While not strictly a hybridization technique, PCR relies heavily on the principles of nucleic acid hybridization. PCR uses short, single-stranded DNA primers that are complementary to specific regions flanking the target DNA sequence. These primers anneal to the target DNA, initiating DNA synthesis by a DNA polymerase. Through repeated cycles of denaturation, annealing, and extension, the target DNA sequence is amplified exponentially. PCR is an indispensable tool in molecular biology for amplifying DNA, cloning genes, and diagnosing infections.
7. Quantitative PCR (qPCR)
qPCR is a variation of PCR that allows for the quantification of the initial amount of target DNA or RNA. The amount of amplified product is monitored in real-time during the PCR reaction, allowing for precise quantification of the target sequence. qPCR is widely used in gene expression studies, disease diagnosis, and forensic science.
Applications of Nucleic Acid Hybridization
The versatility of nucleic acid hybridization has led to its widespread application in various fields:
1. Medical Diagnostics
Nucleic acid hybridization is a cornerstone of numerous diagnostic tests for infectious diseases, genetic disorders, and cancers. Techniques like PCR and microarray analysis are used to detect pathogens, identify genetic mutations, and monitor disease progression.
2. Forensic Science
DNA fingerprinting, based on the principles of nucleic acid hybridization, is a powerful tool in forensic science for identifying individuals and linking suspects to crime scenes.
3. Gene Expression Studies
Nucleic acid hybridization techniques like Northern blotting and microarrays are invaluable tools for studying gene expression levels under various conditions, revealing insights into cellular processes and disease mechanisms.
4. Genetic Engineering
Hybridization is employed in genetic engineering for creating recombinant DNA molecules, where DNA fragments from different sources are joined together. This technology underlies numerous applications, including gene therapy and the production of therapeutic proteins.
5. Phylogenetics
By comparing the sequences of nucleic acids from different organisms, hybridization techniques can reveal evolutionary relationships and build phylogenetic trees. This information is crucial for understanding the diversity of life on Earth.
6. Genomics and Proteomics
High-throughput nucleic acid hybridization techniques, such as microarrays and next-generation sequencing, play a pivotal role in genomics and proteomics, enabling the analysis of entire genomes and proteomes, revealing complex biological systems.
Future Directions
Nucleic acid hybridization continues to evolve with advancements in technology. New developments are focused on increasing the speed, sensitivity, and throughput of hybridization techniques. The use of nanotechnology and novel labeling strategies is paving the way for even more powerful and sophisticated applications. The integration of artificial intelligence and machine learning in analyzing hybridization data holds the potential to further enhance our understanding of complex biological processes. As our understanding of nucleic acid interactions deepens, so too will the impact of nucleic acid hybridization on diverse scientific fields. The inherent specificity and power of this technique ensures its continued importance in the ongoing quest to unravel the mysteries of life.
Latest Posts
Latest Posts
-
Draw The Major Product Of This Elimination
Apr 26, 2025
-
Which Of The Following Is An Example Of B2c E Commerce
Apr 26, 2025
-
Economic Models Do All Of The Following Except
Apr 26, 2025
-
The Book Value Of A Firm Is
Apr 26, 2025
-
A Supersaturated Solution For Nano3 At A Temperature Of 50c
Apr 26, 2025
Related Post
Thank you for visiting our website which covers about Nucleic Acid Hybridization Is Based On The Fact That . We hope the information provided has been useful to you. Feel free to contact us if you have any questions or need further assistance. See you next time and don't miss to bookmark.