Nitrogen And Hydrogen Combine At A High Temperature
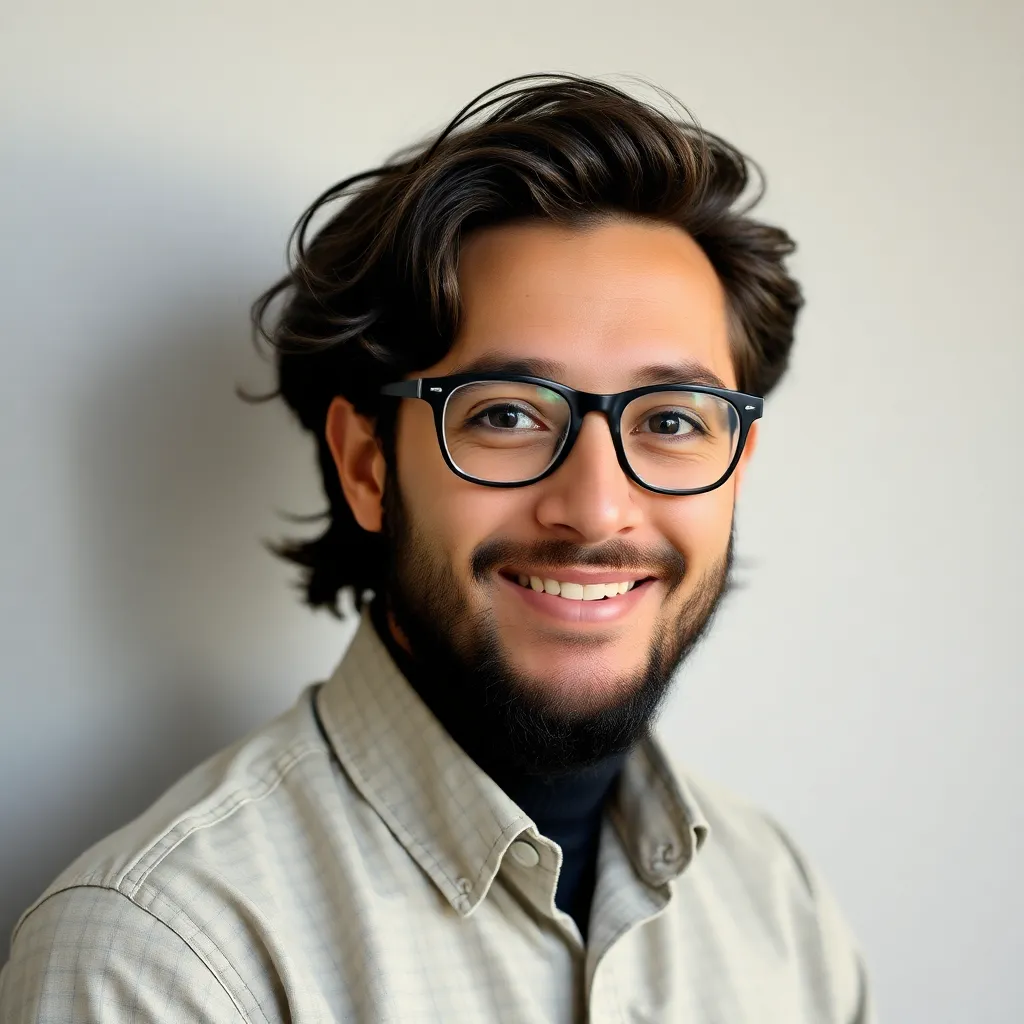
Holbox
May 08, 2025 · 6 min read
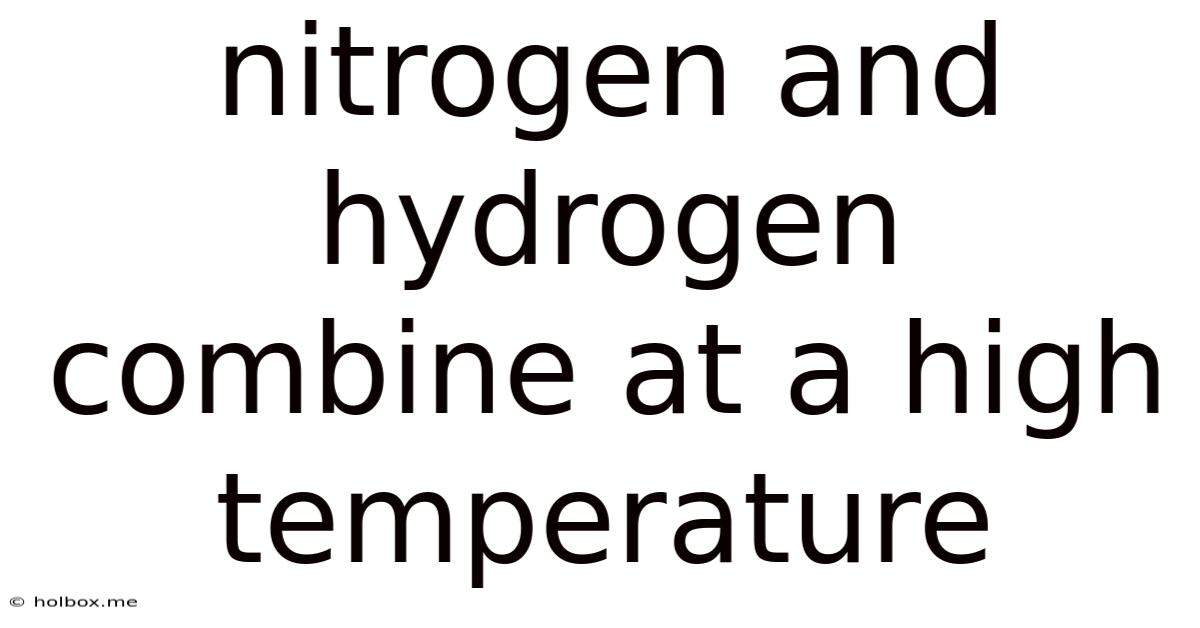
Table of Contents
- Nitrogen And Hydrogen Combine At A High Temperature
- Table of Contents
- The Fiery Dance of Nitrogen and Hydrogen: Exploring the Haber-Bosch Process
- The Chemistry Behind the Reaction: N₂ + 3H₂ ⇌ 2NH₃
- Thermodynamics: An uphill battle
- Kinetics: The speed of the reaction
- The Haber-Bosch Process: A Symphony of Engineering
- 1. Synthesis Gas Production: The Foundation
- 2. Purification: Ensuring Purity
- 3. Compression: High Pressure is Key
- 4. Reaction: The Heart of the Process
- 5. Separation: Harvesting the Ammonia
- 6. Product Purification and Storage: Finishing Touches
- The Impact of the Haber-Bosch Process: Feeding the World
- Environmental Concerns: A Double-Edged Sword
- The Future of Ammonia Synthesis: Towards Sustainability
- Conclusion: A Balancing Act
- Latest Posts
- Related Post
The Fiery Dance of Nitrogen and Hydrogen: Exploring the Haber-Bosch Process
The seemingly simple combination of nitrogen and hydrogen to form ammonia is a cornerstone of modern civilization, yet the process itself is a marvel of chemistry and engineering. This reaction, predominantly carried out through the Haber-Bosch process, involves a high-temperature dance between these two gases, a dance that has profoundly shaped agriculture and industry. Understanding the intricacies of this reaction, including the thermodynamics, kinetics, and industrial applications, reveals a fascinating interplay of scientific principles and practical applications.
The Chemistry Behind the Reaction: N₂ + 3H₂ ⇌ 2NH₃
The fundamental reaction at the heart of ammonia production is the reversible combination of nitrogen gas (N₂) and hydrogen gas (H₂) to yield ammonia (NH₃):
N₂ + 3H₂ ⇌ 2NH₃
This equation elegantly summarizes the stoichiometry: one molecule of nitrogen reacts with three molecules of hydrogen to produce two molecules of ammonia. However, the simplicity of the equation belies the complexity of the reaction itself. Several factors influence the equilibrium and rate of this reaction, making it a challenging yet rewarding area of study.
Thermodynamics: An uphill battle
The formation of ammonia from nitrogen and hydrogen is an exothermic reaction, meaning it releases heat. This is thermodynamically favorable at lower temperatures. However, the reaction is also characterized by a negative change in entropy (ΔS < 0). This means that the reaction proceeds towards a more ordered state (from gaseous reactants to gaseous and liquid products), which is less favorable at lower temperatures.
This inherent conflict between enthalpy (heat) and entropy (disorder) makes the Haber-Bosch process a delicate balance. The Gibbs Free Energy (ΔG), which combines both enthalpy and entropy considerations, dictates the spontaneity of the reaction. At standard temperature and pressure, ΔG is positive, indicating the reaction is not spontaneous. This necessitates the use of high temperatures and pressures to drive the reaction forward.
Kinetics: The speed of the reaction
Even at favorable thermodynamic conditions, the reaction between nitrogen and hydrogen is surprisingly slow. This slowness is primarily due to the strong triple bond in the nitrogen molecule (N₂). This bond requires significant energy to break, creating a high activation energy barrier for the reaction.
To overcome this kinetic limitation, the Haber-Bosch process utilizes a catalyst, typically finely divided iron with promoters like alumina and potassium oxide. The catalyst provides an alternative reaction pathway with a lower activation energy, significantly accelerating the reaction rate without affecting the overall equilibrium. The precise mechanism of catalysis is complex and involves adsorption of reactants onto the catalyst surface, bond breaking and formation, and desorption of the product.
The Haber-Bosch Process: A Symphony of Engineering
The Haber-Bosch process isn't just a chemical reaction; it's a testament to chemical engineering ingenuity. The process involves a series of carefully controlled steps:
1. Synthesis Gas Production: The Foundation
The process begins with the production of synthesis gas, a mixture of hydrogen and nitrogen. This is typically achieved through steam reforming of natural gas (methane), a process that involves reacting methane with steam at high temperatures to produce hydrogen and carbon dioxide. The carbon dioxide is then removed, and the resulting hydrogen is mixed with nitrogen obtained from air separation. The precise composition of the synthesis gas is crucial for optimal ammonia production.
2. Purification: Ensuring Purity
The synthesis gas undergoes extensive purification to remove impurities such as carbon monoxide, sulfur compounds, and other trace elements that can poison the catalyst. This purification step is essential to maintain the catalyst's activity and longevity, ensuring sustained ammonia production.
3. Compression: High Pressure is Key
The purified synthesis gas is then compressed to extremely high pressures, typically ranging from 150 to 350 atmospheres. This high pressure shifts the equilibrium towards ammonia formation, increasing the yield of the reaction. The compression step is energy-intensive but vital for the process's economic viability.
4. Reaction: The Heart of the Process
The compressed synthesis gas is then fed into a reactor containing the iron catalyst. The reactor operates at high temperatures, typically between 400 and 500°C. The reaction is exothermic, and heat is continuously removed to maintain the optimal temperature and prevent catalyst degradation.
5. Separation: Harvesting the Ammonia
The reaction effluent, a mixture of ammonia, unreacted nitrogen and hydrogen, is cooled to condense the ammonia into a liquid. The unreacted gases are recycled back into the reactor to maximize the utilization of the reactants and improve overall efficiency.
6. Product Purification and Storage: Finishing Touches
The liquid ammonia is further purified to remove any residual gases and then stored in pressurized tanks for transport and use. Safety protocols are crucial throughout this process, given the high pressures and toxic nature of some of the reactants and byproducts.
The Impact of the Haber-Bosch Process: Feeding the World
The Haber-Bosch process has had a transformative impact on global food production. Ammonia, the product of this process, is a key ingredient in the production of nitrogen-based fertilizers. These fertilizers have been instrumental in dramatically increasing crop yields over the past century, enabling the world to feed its burgeoning population. Without the Haber-Bosch process, global food production would be drastically reduced, potentially leading to widespread famine.
Environmental Concerns: A Double-Edged Sword
While the Haber-Bosch process has been crucial for feeding the world, it also poses significant environmental challenges. The process is energy-intensive, contributing to greenhouse gas emissions. The production of hydrogen often relies on fossil fuels, further exacerbating the carbon footprint. Furthermore, nitrogen-based fertilizers can lead to eutrophication in water bodies, causing harmful algal blooms and disrupting aquatic ecosystems. The use of ammonia in the production of explosives also raises security concerns.
The Future of Ammonia Synthesis: Towards Sustainability
Researchers are actively exploring ways to make the Haber-Bosch process more sustainable. This includes developing more efficient catalysts, improving reactor design, and exploring alternative energy sources for hydrogen production, such as renewable electricity through electrolysis. The pursuit of a greener ammonia production process is essential to reconcile the critical role of this technology with the imperative to protect the environment. Research focuses on electrocatalytic methods, photocatalytic methods, and biological nitrogen fixation. Improving the efficiency of existing processes through better control and optimization strategies remains a significant area of ongoing research and development.
Conclusion: A Balancing Act
The high-temperature combination of nitrogen and hydrogen, as embodied in the Haber-Bosch process, is a remarkable achievement of chemistry and engineering. It has revolutionized agriculture and continues to support global food security. However, the environmental consequences of this process demand a concerted effort to develop more sustainable methods of ammonia production. The future hinges on finding a balance between meeting the world's food needs and mitigating the environmental impact of this indispensable technology. This ongoing research and development ensure that the fiery dance of nitrogen and hydrogen continues to benefit humanity while minimizing its environmental footprint. The quest for a sustainable future necessitates a commitment to innovation and a continuous refinement of this crucial industrial process.
Latest Posts
Related Post
Thank you for visiting our website which covers about Nitrogen And Hydrogen Combine At A High Temperature . We hope the information provided has been useful to you. Feel free to contact us if you have any questions or need further assistance. See you next time and don't miss to bookmark.