Multiple Proteins Are Involved In Dna Replication
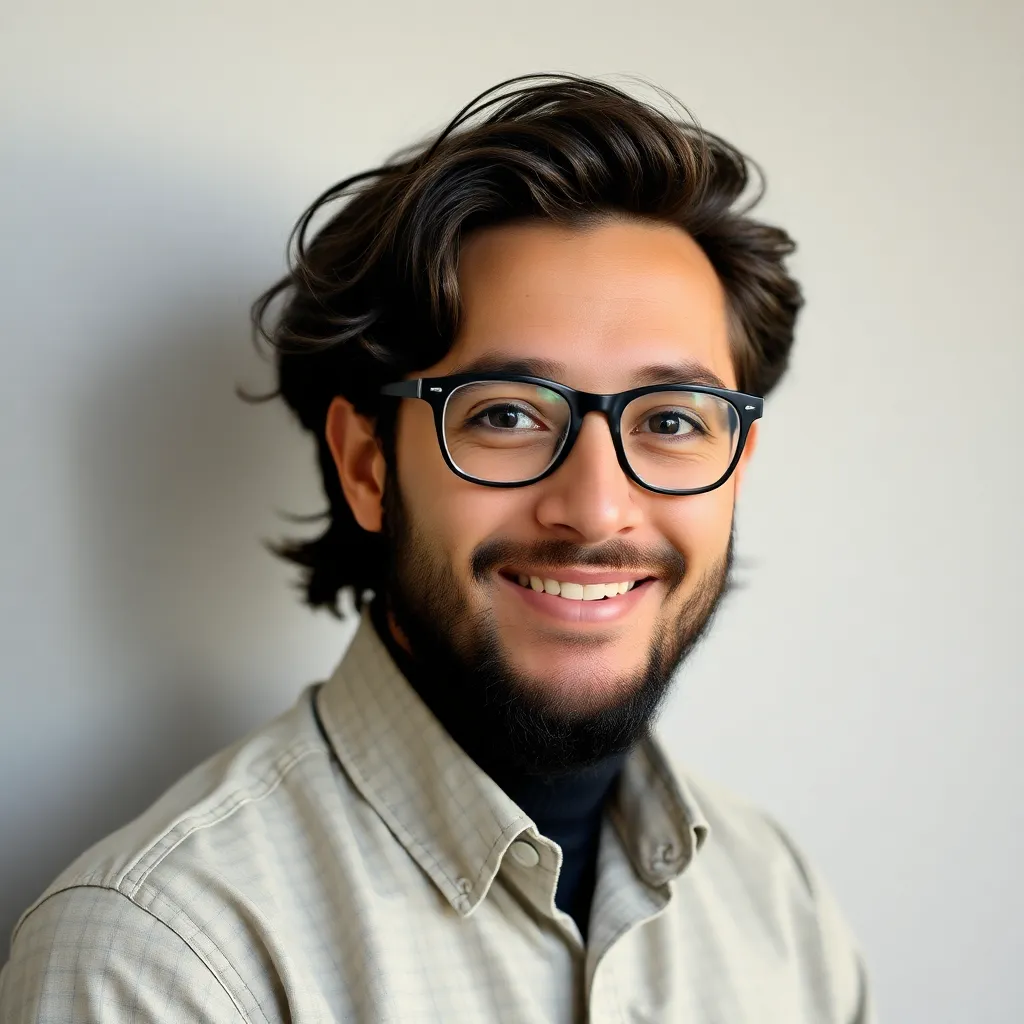
Holbox
May 08, 2025 · 7 min read
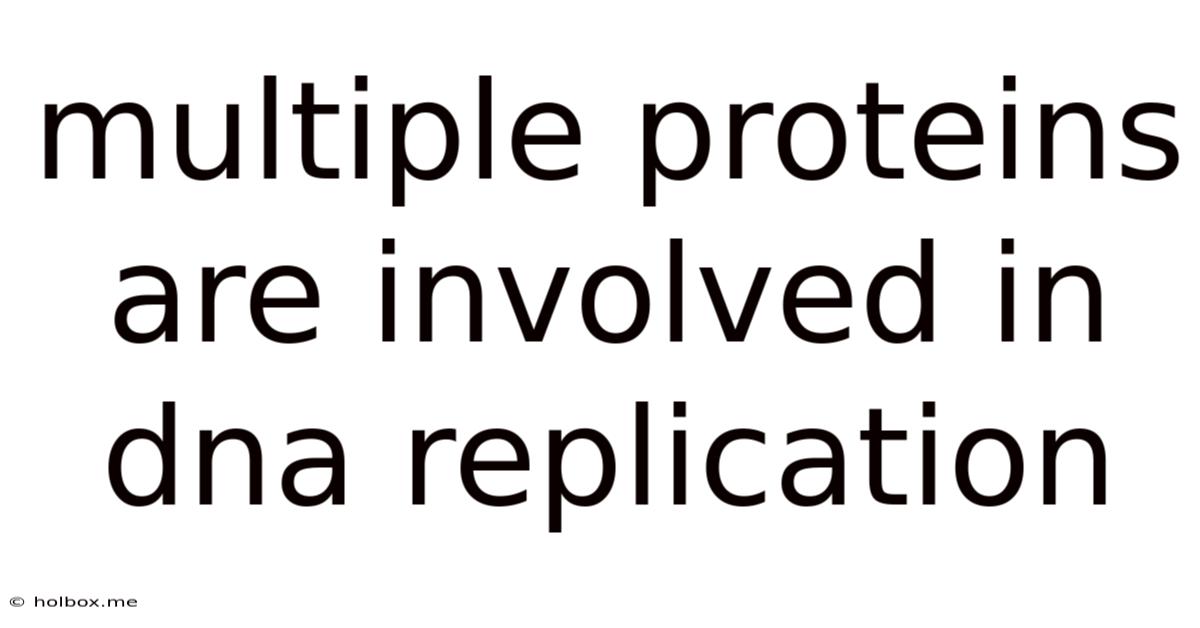
Table of Contents
- Multiple Proteins Are Involved In Dna Replication
- Table of Contents
- Multiple Proteins Orchestrate the Intricate Dance of DNA Replication
- The Key Players: Enzymes and Accessory Proteins
- 1. Helicases: Unwinding the Double Helix
- 2. Topoisomerases: Relieving Torsional Stress
- 3. Single-Strand Binding Proteins (SSBs): Stabilizing Single-Stranded DNA
- 4. Primase: Synthesizing RNA Primers
- 5. DNA Polymerases: Synthesizing New DNA Strands
- 6. Sliding Clamps: Enhancing Polymerase Processivity
- 7. Ligase: Joining Okazaki Fragments
- 8. Telomerase: Maintaining Telomeres
- The Coordinated Effort: A Symphony of Interactions
- The Replication Fork: A Dynamic Structure
- Regulation and Fidelity: Ensuring Accuracy
- Differences between Prokaryotic and Eukaryotic Replication
- Clinical Significance: The Impact of Replication Errors
- Conclusion: An Ongoing Area of Research
- Latest Posts
- Related Post
Multiple Proteins Orchestrate the Intricate Dance of DNA Replication
DNA replication, the process by which a cell creates an exact copy of its DNA before cell division, is a marvel of biological precision. Far from being a simple duplication process, it's a highly orchestrated molecular ballet involving a complex interplay of numerous proteins. Each protein plays a specific and crucial role, ensuring fidelity and efficiency in the creation of new DNA strands. A failure in even one protein's function can lead to catastrophic consequences, resulting in mutations and genomic instability. This article delves into the diverse cast of proteins involved, exploring their individual functions and their collaborative roles in the intricate mechanism of DNA replication.
The Key Players: Enzymes and Accessory Proteins
DNA replication is a multi-step process requiring a diverse array of enzymes and accessory proteins. These can be broadly categorized based on their roles:
1. Helicases: Unwinding the Double Helix
The DNA double helix, a stable structure held together by hydrogen bonds between base pairs, must first be unwound to allow access to the individual strands. This crucial step is carried out by helicases, motor proteins that use ATP hydrolysis to break the hydrogen bonds and separate the two parental strands, creating a replication fork. Different helicases may be involved depending on the organism and the specific replication context. The unwinding process introduces torsional stress ahead of the replication fork, which is relieved by another group of proteins:
2. Topoisomerases: Relieving Torsional Stress
As the helicases unwind the DNA, the remaining DNA strands become increasingly twisted, leading to supercoiling. Topoisomerases are enzymes that alleviate this supercoiling by cutting and rejoining DNA strands. They prevent the build-up of torsional stress, ensuring the smooth progression of the replication fork. Type I topoisomerases cut one strand, while Type II topoisomerases cut both strands, introducing or removing supercoils as needed.
3. Single-Strand Binding Proteins (SSBs): Stabilizing Single-Stranded DNA
Once separated, the single-stranded DNA (ssDNA) is vulnerable to degradation and secondary structure formation. Single-strand binding proteins (SSBs) bind to the ssDNA, preventing it from reannealing with its complementary strand or forming hairpin loops. SSBs maintain the ssDNA in a conformation suitable for replication, acting as a scaffold for other proteins involved in the process. Their binding is dynamic, allowing access for the DNA polymerase and other enzymes.
4. Primase: Synthesizing RNA Primers
DNA polymerases, the enzymes responsible for synthesizing new DNA strands, cannot initiate DNA synthesis de novo. They require a pre-existing 3'-OH group to add nucleotides to. This crucial role is fulfilled by primase, an RNA polymerase that synthesizes short RNA primers complementary to the template DNA strand. These RNA primers provide the 3'-OH group necessary for DNA polymerase to begin synthesis. Primase often functions as part of a larger complex called the primosome.
5. DNA Polymerases: Synthesizing New DNA Strands
DNA polymerases are the workhorses of DNA replication, responsible for adding nucleotides to the 3'-OH end of the growing DNA strand. Different DNA polymerases have specialized roles:
- DNA Polymerase III (in prokaryotes): The primary polymerase responsible for the bulk of DNA synthesis. It possesses high processivity, meaning it can add many nucleotides before dissociating from the template.
- DNA Polymerase I (in prokaryotes): Removes RNA primers and replaces them with DNA. It also possesses 5' to 3' exonuclease activity to remove the RNA primers.
- Various eukaryotic DNA polymerases: Eukaryotes have multiple DNA polymerases with specialized functions in leading and lagging strand synthesis, DNA repair, and mitochondrial DNA replication. Examples include DNA polymerase α, δ, and ε.
DNA polymerases exhibit remarkable fidelity, incorporating the correct nucleotides with high accuracy. They possess a proofreading function, which is usually a 3' to 5' exonuclease activity that removes incorrectly incorporated nucleotides, minimizing errors during DNA replication.
6. Sliding Clamps: Enhancing Polymerase Processivity
DNA polymerases, while highly efficient, tend to dissociate from the template strand frequently. Sliding clamps are ring-shaped proteins that encircle the DNA and enhance the processivity of DNA polymerases. They act as a “clamp” to keep the polymerase attached to the DNA template, allowing for continuous synthesis. The clamp loader is a protein complex responsible for loading the sliding clamp onto the DNA.
7. Ligase: Joining Okazaki Fragments
DNA replication on the lagging strand occurs discontinuously, generating short fragments called Okazaki fragments. These fragments are joined together by DNA ligase, an enzyme that catalyzes the formation of a phosphodiester bond between the 3'-OH end of one Okazaki fragment and the 5'-phosphate end of the next, creating a continuous strand.
8. Telomerase: Maintaining Telomeres
Telomeres, repetitive DNA sequences at the ends of chromosomes, are crucial for maintaining chromosome integrity. During replication, the lagging strand cannot be fully replicated, leading to telomere shortening. Telomerase, a ribonucleoprotein enzyme, extends telomeres by adding repetitive DNA sequences, preventing telomere erosion and maintaining chromosome stability. Its activity is tightly regulated, and its dysregulation is implicated in aging and cancer.
The Coordinated Effort: A Symphony of Interactions
The proteins involved in DNA replication don't operate in isolation; they form a highly coordinated molecular machine, a replisome, that ensures efficient and accurate DNA replication. The replisome comprises all the enzymes and proteins involved, working together in a precise and regulated manner.
The Replication Fork: A Dynamic Structure
The replication fork is the site where the DNA double helix unwinds and new DNA strands are synthesized. It's a dynamic structure involving a complex interplay of proteins. Helicases actively unwind the DNA, creating single-stranded regions, while SSBs stabilize these regions, preventing them from reannealing. Primase synthesizes RNA primers, providing starting points for DNA polymerase, which extends the primers to synthesize new DNA strands. Sliding clamps enhance polymerase processivity, ensuring continuous synthesis. Topoisomerases relieve torsional stress ahead of the fork, preventing DNA breakage. The coordination of these activities ensures that DNA replication proceeds smoothly and efficiently.
Regulation and Fidelity: Ensuring Accuracy
The process of DNA replication is tightly regulated to ensure high fidelity. This includes the proofreading activity of DNA polymerases, which corrects errors during nucleotide incorporation. Mismatch repair systems, involving a separate set of proteins, detect and repair mismatched base pairs that escape the proofreading mechanism. These quality control mechanisms maintain the integrity of the genome, minimizing the occurrence of mutations.
Differences between Prokaryotic and Eukaryotic Replication
While the fundamental principles of DNA replication are conserved across all organisms, there are significant differences between prokaryotic and eukaryotic replication:
- Number of origins of replication: Prokaryotes typically have a single origin of replication, whereas eukaryotes have multiple origins of replication on each chromosome, allowing for faster replication.
- DNA polymerases: Prokaryotes have fewer types of DNA polymerases compared to eukaryotes, which possess a more diverse set of DNA polymerases with specialized functions.
- Complexity of the replisome: The eukaryotic replisome is significantly more complex than the prokaryotic replisome, reflecting the greater complexity of eukaryotic genomes.
- Telomere replication: Eukaryotic chromosomes have telomeres, which require telomerase for their replication, a mechanism absent in prokaryotes.
Clinical Significance: The Impact of Replication Errors
Errors in DNA replication can have profound consequences, leading to mutations and genomic instability. These errors can arise from various factors, including defects in DNA polymerases, impaired mismatch repair, and telomere dysfunction. Such errors can contribute to the development of various diseases, including cancer. The study of DNA replication is therefore crucial for understanding the mechanisms of disease and developing therapeutic strategies.
Conclusion: An Ongoing Area of Research
The process of DNA replication is a remarkably intricate and highly regulated process, involving a large number of proteins working in concert to faithfully duplicate the genome. While significant progress has been made in understanding the individual roles of these proteins and their interactions, research continues to unravel the complexities of this fundamental biological process. Further research into the mechanisms of DNA replication is crucial for understanding genome stability, disease pathogenesis, and developing new therapeutic approaches. The exploration of this fascinating molecular machinery remains a vibrant and essential area of scientific investigation.
Latest Posts
Related Post
Thank you for visiting our website which covers about Multiple Proteins Are Involved In Dna Replication . We hope the information provided has been useful to you. Feel free to contact us if you have any questions or need further assistance. See you next time and don't miss to bookmark.