Label The Parts Of The Dna Replication Fork
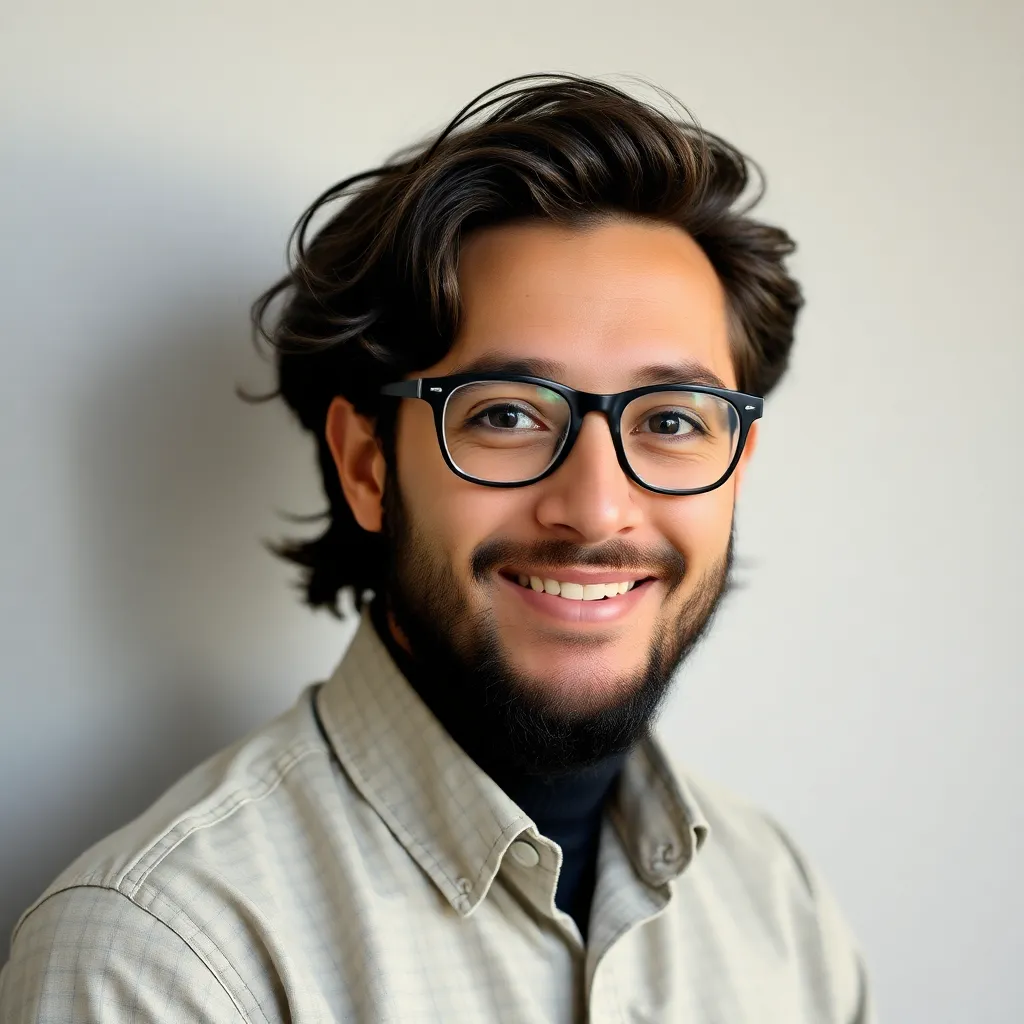
Holbox
May 13, 2025 · 7 min read
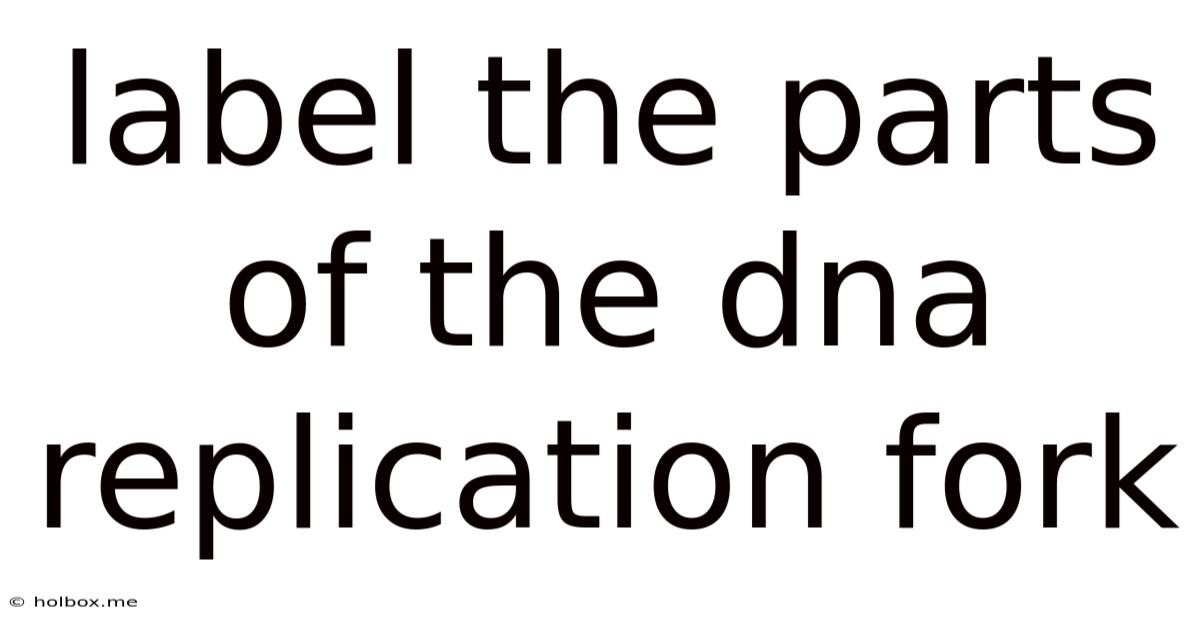
Table of Contents
- Label The Parts Of The Dna Replication Fork
- Table of Contents
- Labeling the Parts of the DNA Replication Fork: A Deep Dive
- The Key Players at the Replication Fork
- 1. Parental DNA (Template Strands):
- 2. DNA Helicase: The Unwinding Enzyme
- 3. Single-Stranded Binding Proteins (SSBs): Stabilizing the Templates
- 4. Topoisomerase (DNA Gyrase): Relieving Torsional Stress
- 5. Primase: Providing the Starting Point
- 6. DNA Polymerase: The Master Builder
- 7. DNA Ligase: Joining the Fragments
- 8. Sliding Clamp: Enhancing Processivity
- 9. Clamp Loader: Assembling the Sliding Clamp
- Understanding the Replication Fork Dynamics
- Beyond the Basics: Variations and Further Exploration
- Conclusion: The Replication Fork – A Molecular Masterpiece
- Latest Posts
- Related Post
Labeling the Parts of the DNA Replication Fork: A Deep Dive
DNA replication, the fundamental process by which cells duplicate their genetic material, is a marvel of biological engineering. Central to this process is the replication fork, a Y-shaped structure where the double-stranded DNA helix unwinds and separates, creating two single-stranded templates for new DNA synthesis. Understanding the components and their functions within this dynamic structure is crucial for grasping the intricacies of DNA replication. This article provides a detailed exploration of the replication fork, meticulously labeling its key players and explaining their roles.
The Key Players at the Replication Fork
The replication fork isn't just a static structure; it's a bustling hub of enzymatic activity, with numerous proteins working in concert to ensure accurate and efficient DNA duplication. Let's delve into the major components:
1. Parental DNA (Template Strands):
This is the starting point – the original double-stranded DNA molecule that needs to be replicated. The parental DNA consists of two antiparallel strands: a leading strand and a lagging strand. These strands serve as templates for the synthesis of new complementary strands. The leading strand is synthesized continuously in the 5' to 3' direction, while the lagging strand is synthesized discontinuously in short fragments called Okazaki fragments. The unwinding of the parental DNA creates the replication fork itself.
2. DNA Helicase: The Unwinding Enzyme
DNA helicase is a crucial enzyme that plays the role of the “unzipper.” It actively unwinds the parental DNA double helix at the replication fork, breaking the hydrogen bonds between the complementary base pairs (adenine with thymine, and guanine with cytosine). This unwinding generates the single-stranded DNA templates necessary for replication. The helicase moves along the DNA in a 5' to 3' direction, creating a single-stranded region ahead of the replication fork. Its continuous action ensures a constant supply of template DNA.
3. Single-Stranded Binding Proteins (SSBs): Stabilizing the Templates
Once the DNA strands separate, they're susceptible to re-annealing (re-pairing). To prevent this, single-stranded binding proteins (SSBs) bind to the separated strands, keeping them apart and stable. These proteins coat the single-stranded DNA, protecting it from degradation and providing a suitable template for the DNA polymerase enzymes. SSBs are essential for maintaining the integrity and accessibility of the template strands during replication.
4. Topoisomerase (DNA Gyrase): Relieving Torsional Stress
As the DNA helicase unwinds the helix, torsional stress builds up ahead of the replication fork. This stress, manifested as supercoiling, can hinder the unwinding process. Topoisomerases, such as DNA gyrase in prokaryotes, are enzymes that alleviate this stress by temporarily breaking and rejoining DNA strands. They essentially relieve the supercoils, allowing the replication fork to move smoothly along the DNA molecule. Without topoisomerases, the replication process would be significantly hampered or even stalled.
5. Primase: Providing the Starting Point
DNA polymerase, the enzyme that synthesizes new DNA strands, can only add nucleotides to an existing 3'-OH group. It cannot initiate DNA synthesis de novo. Therefore, primase, an RNA polymerase, plays a critical role by synthesizing short RNA primers. These primers provide the necessary 3'-OH group that DNA polymerase requires to begin adding nucleotides. Primase synthesizes short RNA sequences complementary to the DNA template, creating a starting point for DNA synthesis on both the leading and lagging strands.
6. DNA Polymerase: The Master Builder
DNA polymerase is the workhorse of DNA replication, responsible for the actual synthesis of new DNA strands. Several different DNA polymerases exist, each with specialized roles. The most important is DNA polymerase III in prokaryotes and DNA polymerase δ and ε in eukaryotes, which have high processivity—meaning they can add many nucleotides to a growing strand without detaching. DNA polymerase adds nucleotides to the 3' end of the primer, extending the strand in the 5' to 3' direction. This process ensures the accurate replication of the DNA sequence by following the base-pairing rules (A with T, and G with C).
7. DNA Ligase: Joining the Fragments
On the lagging strand, DNA synthesis occurs in short fragments, called Okazaki fragments. Each Okazaki fragment is initiated by an RNA primer and extended by DNA polymerase. However, these fragments need to be joined together to form a continuous strand. DNA ligase is the enzyme that catalyzes the formation of phosphodiester bonds between the adjacent Okazaki fragments, sealing the gaps and creating a complete, continuous lagging strand. This essential step ensures the integrity and stability of the newly synthesized DNA.
8. Sliding Clamp: Enhancing Processivity
DNA polymerase's processivity (the ability to stay attached to the template and synthesize long stretches of DNA) is greatly enhanced by the sliding clamp. This ring-shaped protein encircles the DNA and keeps the polymerase firmly attached to the template strand, preventing premature dissociation. This increases the efficiency of DNA replication by allowing the polymerase to synthesize longer stretches of DNA continuously.
9. Clamp Loader: Assembling the Sliding Clamp
The clamp loader is a protein complex that loads the sliding clamp onto the DNA. This is a crucial step, as the clamp loader ensures that the sliding clamp is positioned correctly at the replication fork, enabling the efficient and accurate functioning of DNA polymerase. The clamp loader works in concert with other proteins to ensure seamless DNA replication.
Understanding the Replication Fork Dynamics
The replication fork isn't a static structure; it's a highly dynamic and coordinated assembly of proteins. The continuous unwinding of the parental DNA by helicase creates a constant influx of single-stranded DNA, which is then processed by the other enzymes to produce new DNA strands. This coordinated action ensures accurate and efficient replication.
The leading strand synthesis is relatively straightforward—continuous and in the 5' to 3' direction. The lagging strand synthesis, however, is more complex, involving the discontinuous synthesis of Okazaki fragments and subsequent joining by DNA ligase. This difference arises from the antiparallel nature of the DNA strands and the inherent directionality of DNA polymerase.
The whole process is tightly regulated, preventing errors and ensuring the fidelity of DNA replication. Proofreading mechanisms within DNA polymerase help correct errors during synthesis, minimizing mutations. Furthermore, various checkpoints exist throughout the replication process to ensure that DNA replication is complete and accurate before the cell proceeds to cell division.
Beyond the Basics: Variations and Further Exploration
While the above description provides a foundational understanding of the replication fork components, the specifics can vary across different organisms. Prokaryotic and eukaryotic replication forks, for example, exhibit differences in the specific proteins involved and the overall process dynamics. Eukaryotic replication is notably more complex due to the presence of nucleosomes (DNA packaged around histone proteins). The replication machinery must navigate and reassemble these nucleosomes during replication.
Furthermore, research continually reveals new details about the intricate workings of the replication fork. Studies are ongoing to better understand the precise mechanisms of protein interactions, regulatory pathways, and the resolution of replication stress, which can lead to DNA damage and genomic instability.
Conclusion: The Replication Fork – A Molecular Masterpiece
The replication fork is a truly remarkable structure, showcasing the exquisite precision and efficiency of cellular processes. The intricate interplay of enzymes and proteins at the replication fork ensures that DNA is accurately replicated, passing on genetic information with remarkable fidelity. Understanding the components and their functions is fundamental to comprehending the mechanisms of heredity, and appreciating the complexity of life at the molecular level. Continued research into the replication fork promises further insights into this fundamental process and its implications for cell biology, genetics, and human health. The detailed labeling and explanation of the components provided here serve as a valuable resource for anyone wishing to delve deeper into this fascinating area of molecular biology.
Latest Posts
Related Post
Thank you for visiting our website which covers about Label The Parts Of The Dna Replication Fork . We hope the information provided has been useful to you. Feel free to contact us if you have any questions or need further assistance. See you next time and don't miss to bookmark.