Label Each Element Involved In Bacterial Transcription In The Figure.
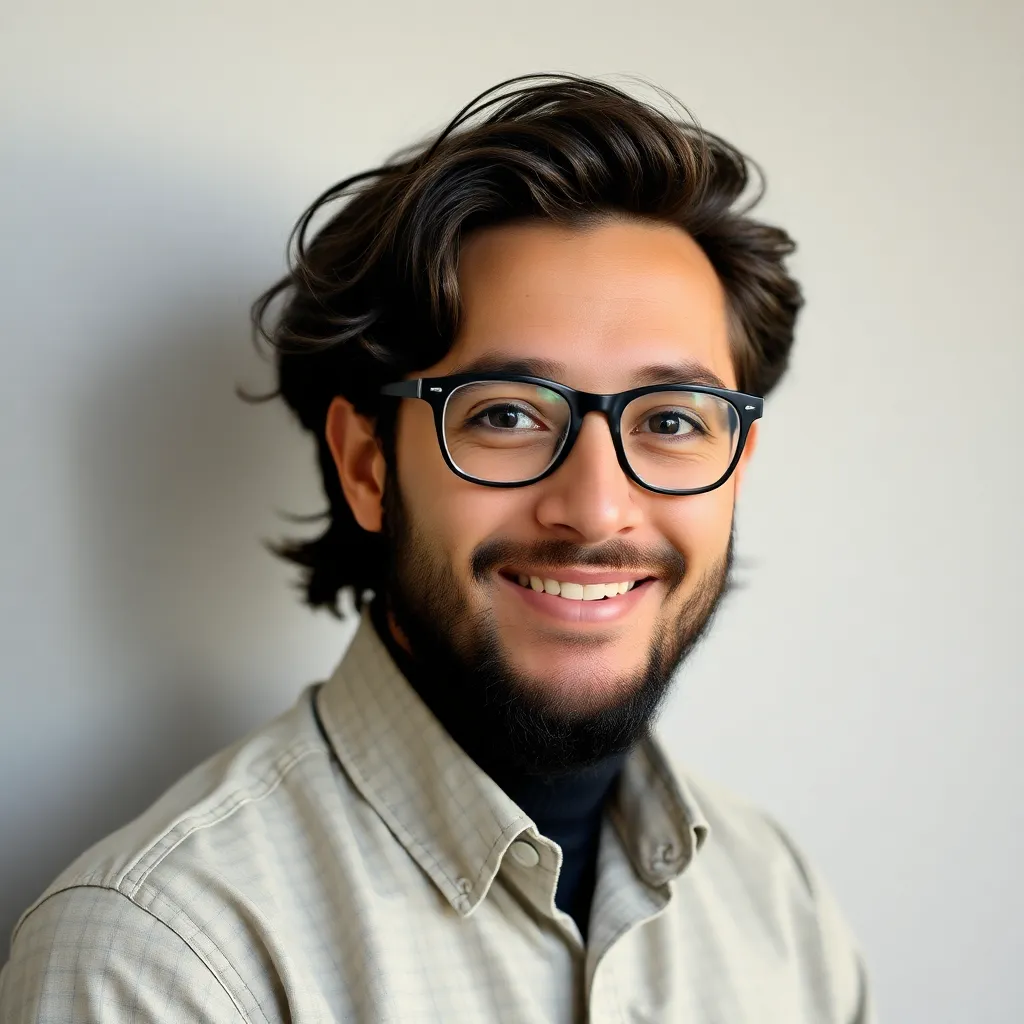
Holbox
May 12, 2025 · 6 min read
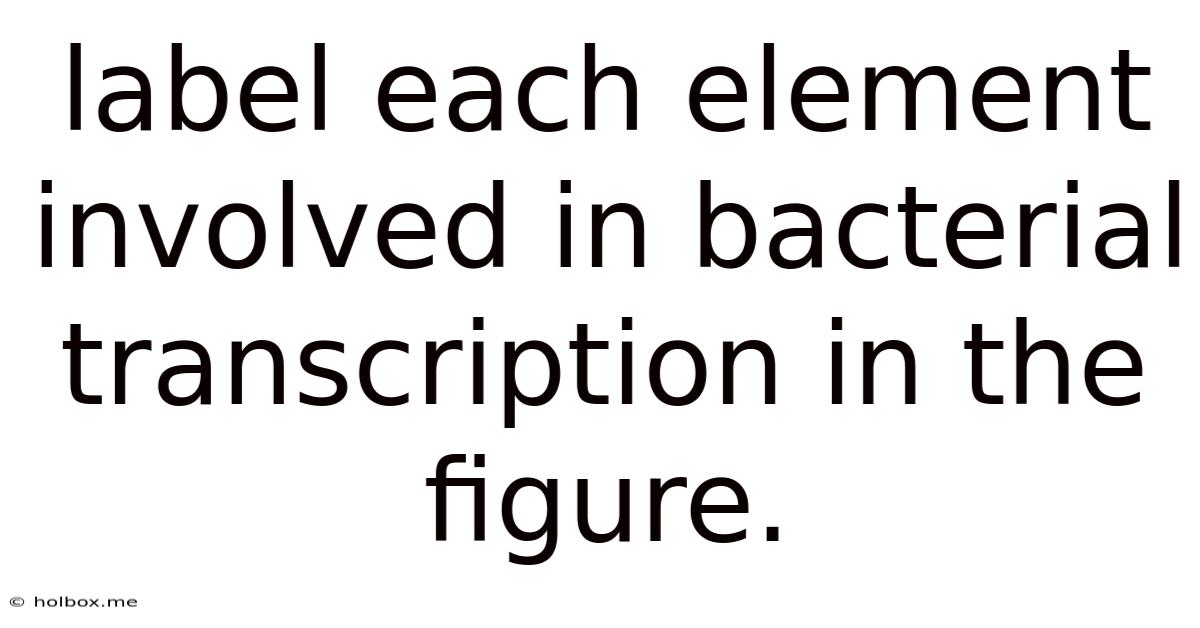
Table of Contents
- Label Each Element Involved In Bacterial Transcription In The Figure.
- Table of Contents
- Labeling the Elements Involved in Bacterial Transcription: A Deep Dive
- The Core Players: RNA Polymerase and the Promoter
- 1. The Core Enzyme:
- 2. The Sigma Factor (σ):
- The Promoter Region:
- Transcription Initiation: From Binding to Bubble Formation
- Transcription Elongation: Building the RNA Transcript
- Transcription Termination: Ending the RNA Synthesis
- 1. Rho-independent Termination:
- 2. Rho-dependent Termination:
- Post-Transcriptional Modifications
- Conclusion: A Complex Yet Precise Process
- Latest Posts
- Latest Posts
- Related Post
Labeling the Elements Involved in Bacterial Transcription: A Deep Dive
Bacterial transcription, the process of synthesizing RNA from a DNA template, is a fundamental process crucial for bacterial life. Understanding its intricate mechanisms is essential for comprehending bacterial gene regulation and developing effective antimicrobial strategies. This article provides a detailed examination of the key elements involved in bacterial transcription, illustrated through a conceptual figure (which I will describe textually since I cannot display images directly). We will meticulously label each component and explore its function in this vital cellular process.
The Core Players: RNA Polymerase and the Promoter
The central player in bacterial transcription is RNA polymerase (RNAP). This enzyme is a molecular machine responsible for unwinding the DNA double helix, incorporating ribonucleotides to synthesize RNA, and proofreading the newly synthesized RNA molecule. Bacterial RNAP is a holoenzyme, meaning it's a multi-subunit complex consisting of:
1. The Core Enzyme:
-
α (alpha) subunits (two): These subunits are involved in enzyme assembly and interaction with regulatory proteins. They play a crucial role in promoter recognition and binding. They're essential for the stability and correct assembly of the RNAP holoenzyme.
-
β (beta) subunit: This subunit is the primary catalytic subunit, directly responsible for the polymerization of ribonucleotides to form the RNA chain. It’s the active site for nucleotide addition.
-
β' (beta-prime) subunit: This subunit is also crucial for catalytic activity. It contributes to the DNA binding and overall enzyme stability, working in concert with the β subunit.
-
ω (omega) subunit: While its exact role isn't fully elucidated, the ω subunit helps in the assembly and stabilization of the core enzyme.
2. The Sigma Factor (σ):
The sigma factor (σ) is a crucial regulatory subunit that is not part of the core enzyme, but rather a temporary addition. It's essential for promoter recognition. The sigma factor directs the core enzyme to specific DNA sequences called promoters, thus determining which genes are transcribed. Different sigma factors recognize different promoter sequences, allowing bacteria to respond to various environmental stimuli by altering which genes are expressed. For example, E. coli has multiple sigma factors (σ<sup>70</sup>, σ<sup>32</sup>, σ<sup>S</sup>, etc.) each responding to specific cellular situations.
The Promoter Region:
The promoter is a specific DNA sequence located upstream of the transcription start site. It acts as the binding site for RNAP holoenzyme. Key elements within the promoter include:
-
-10 region (Pribnow box): This sequence, typically TATAAT, is highly conserved and is located approximately 10 base pairs upstream of the transcription start site (+1). It is the site of initial unwinding of the DNA double helix.
-
-35 region: This sequence, typically TTGACA, is located approximately 35 base pairs upstream of the transcription start site. It contributes to the initial binding of the RNAP holoenzyme. The precise sequence varies depending on the specific promoter and the associated sigma factor.
-
Spacer region: The region between the -35 and -10 elements, typically 17 bp long, is crucial for proper promoter function. Variations in spacer length can affect promoter strength.
Transcription Initiation: From Binding to Bubble Formation
Transcription initiation involves several steps:
-
Holoenzyme Binding: The RNAP holoenzyme, containing the core enzyme and the sigma factor, binds loosely to the promoter region. The sigma factor plays a critical role in this initial recognition and binding.
-
Closed Complex Formation: The RNAP holoenzyme forms a closed complex with the DNA, where the DNA double helix remains intact.
-
Open Complex Formation: The RNAP holoenzyme unwinds approximately 12-14 base pairs of the DNA double helix around the -10 region, creating a transcription bubble. This unwinding requires energy and is mediated by the RNAP enzyme. This open complex is now ready for RNA synthesis.
-
Initiation of RNA Synthesis: The RNAP holoenzyme incorporates the first ribonucleotide, typically a purine (A or G), at the +1 site. This marks the beginning of the RNA transcript. The first few nucleotides are often incorporated rather inefficiently; this is termed "abortive initiation."
Transcription Elongation: Building the RNA Transcript
Once the initial few nucleotides are incorporated, the sigma factor dissociates from the RNAP core enzyme. The core enzyme continues to move along the DNA template, unwinding the DNA ahead and rewinding it behind, synthesizing the RNA molecule. During this elongation phase:
-
DNA unwinding and rewinding: RNAP continuously unwinds a small region of DNA ahead of it, creating a transcription bubble. Behind the bubble, the DNA strands are rewound.
-
RNA synthesis: The RNAP core enzyme uses the DNA template strand to synthesize RNA in the 5' to 3' direction. The RNA molecule is complementary to the template strand but identical to the non-template (coding) strand, except for uracil (U) in place of thymine (T).
-
Proofreading: RNAP possesses limited proofreading capabilities, correcting some incorporation errors. However, its error rate is significantly higher than DNA polymerase.
-
Transcription pausing and termination signals: Transcription elongation is not always continuous. The process can pause at certain sequences or be terminated by specific sequences called termination signals.
Transcription Termination: Ending the RNA Synthesis
Transcription termination signals the end of RNA synthesis. Bacteria employ two major mechanisms for transcription termination:
1. Rho-independent Termination:
This mechanism involves specific sequences within the DNA that promote the formation of a stable hairpin structure in the newly synthesized RNA molecule. This hairpin structure causes the RNA polymerase to pause, and the weak interactions between the RNA and the DNA template strand are disrupted, releasing the RNA molecule and terminating transcription. The hairpin structure is typically followed by a string of uracil residues in the RNA transcript which further destabilize the RNA-DNA hybrid.
2. Rho-dependent Termination:
This mechanism involves the protein Rho (ρ) factor. Rho is a hexameric ATPase which binds to the RNA molecule near the 3' end and tracks along the RNA molecule towards the RNAP. When it catches up to the paused RNAP, it causes the unwinding and dissociation of the RNA-DNA hybrid, terminating transcription. Rho-dependent termination often involves a rut site (rho utilization site) on the RNA transcript, a region less prone to secondary structure formation, allowing Rho to easily bind and track.
Post-Transcriptional Modifications
Once the RNA transcript is synthesized, it can undergo various post-transcriptional modifications before it can be translated into protein. These modifications can include:
-
5' capping: Addition of a modified nucleotide at the 5' end of the RNA molecule to protect it from degradation. This is not typical in bacteria, unlike eukaryotes.
-
3' polyadenylation: Addition of a poly(A) tail at the 3' end of the RNA molecule to increase stability and signal translation. Again, this is less common in bacteria compared to eukaryotes.
-
RNA splicing: Removal of introns (non-coding sequences) from the RNA molecule. Bacteria rarely have introns, unlike eukaryotes. However, some bacterial RNAs may undergo self-splicing.
-
RNA editing: Chemical changes in the RNA sequence. This is less common than in some eukaryotes.
Conclusion: A Complex Yet Precise Process
Bacterial transcription is a remarkably complex and tightly regulated process. The coordinated action of RNA polymerase, sigma factors, and various termination mechanisms ensures the precise synthesis of RNA molecules, which are essential for protein synthesis and gene regulation. Further investigation into the intricate mechanisms of bacterial transcription will continue to reveal insights into bacterial biology, contributing to advancements in diverse fields, from antibiotic development to synthetic biology. A thorough understanding of the elements involved, as detailed above, provides a solid foundation for deeper exploration into this crucial biological process.
Latest Posts
Latest Posts
-
How Many Weeks Is 47 Days
May 20, 2025
-
How Many Days Are In 13 Weeks
May 20, 2025
-
How Many Weeks In 84 Days
May 20, 2025
-
5000 Square Feet In Square Meters
May 20, 2025
-
25 Days Is How Many Weeks
May 20, 2025
Related Post
Thank you for visiting our website which covers about Label Each Element Involved In Bacterial Transcription In The Figure. . We hope the information provided has been useful to you. Feel free to contact us if you have any questions or need further assistance. See you next time and don't miss to bookmark.