Interstitial Solid Solution C Solute In Fe
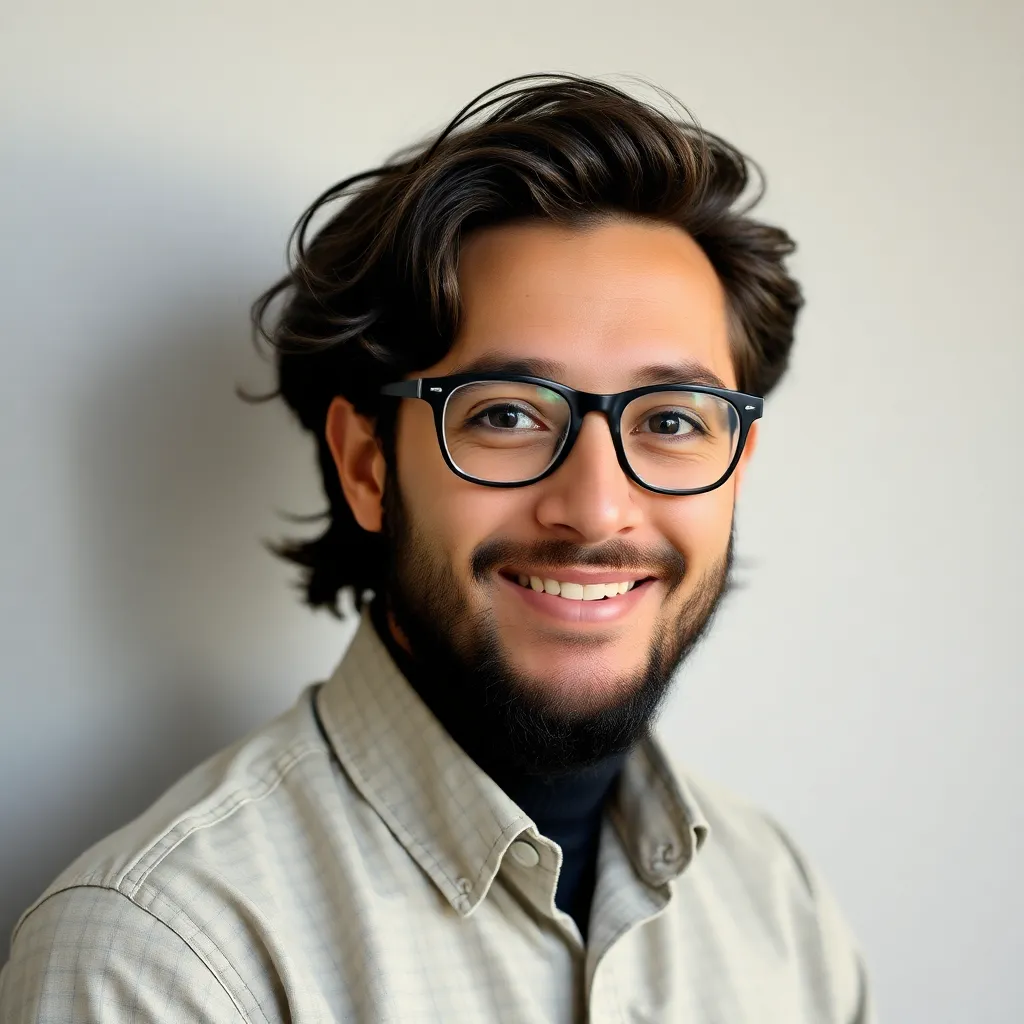
Holbox
Mar 13, 2025 · 7 min read

Table of Contents
- Interstitial Solid Solution C Solute In Fe
- Table of Contents
- Interstitial Solid Solution: C Solute in Fe
- Understanding Interstitial Solid Solutions
- Iron's Crystal Structures: BCC and FCC
- Body-Centered Cubic (BCC) Iron (α-Iron)
- Face-Centered Cubic (FCC) Iron (γ-Iron)
- Carbon's Influence on Iron's Properties
- Strengthening Mechanisms
- Influence on Phase Transformations
- Influence on Mechanical Properties
- Phase Diagrams and their Importance
- Applications of C-Fe Interstitial Solid Solutions
- Advanced Techniques for Characterizing C in Fe
- Future Research Directions
- Latest Posts
- Latest Posts
- Related Post
Interstitial Solid Solution: C Solute in Fe
Iron (Fe) and carbon (C) form a crucial system in materials science, underpinning the vast field of ferrous metallurgy. Understanding their interaction, particularly in the context of interstitial solid solutions, is fundamental to designing and optimizing steel properties. This article delves deep into the intricacies of interstitial solid solutions where carbon acts as the solute within an iron matrix, examining its effects on crystal structure, mechanical properties, and phase transformations.
Understanding Interstitial Solid Solutions
A solid solution is a homogeneous mixture where one element (the solute) dissolves within the crystal structure of another (the solvent). There are two main types: substitutional and interstitial. In substitutional solid solutions, the solute atoms replace the solvent atoms in the lattice. Interstitial solid solutions, however, occur when the solute atoms are significantly smaller than the solvent atoms and occupy the interstitial sites – the spaces between the solvent atoms in the crystal lattice. This is the case with carbon in iron.
Carbon atoms, being significantly smaller than iron atoms, readily occupy the octahedral and tetrahedral interstitial sites within the iron crystal structure. This ability to fit into the spaces between iron atoms is crucial for the formation of interstitial solid solutions and significantly influences the properties of the resulting material.
Iron's Crystal Structures: BCC and FCC
Iron exhibits two main allotropic forms, each possessing a distinct crystal structure:
Body-Centered Cubic (BCC) Iron (α-Iron)
At lower temperatures (below 912°C), iron adopts a body-centered cubic (BCC) structure. In this structure, iron atoms are positioned at the corners and the center of a cube. The interstitial sites within the BCC structure are relatively small, limiting the amount of carbon that can dissolve interstitially. This is why the solubility of carbon in BCC iron is quite low.
Face-Centered Cubic (FCC) Iron (γ-Iron)
Above 912°C, iron transforms to a face-centered cubic (FCC) structure. In the FCC structure, iron atoms are located at each corner and the center of each face of the cube. This structure offers larger interstitial sites compared to BCC iron, allowing for a significantly higher solubility of carbon. The increased solubility of carbon in γ-iron has profound implications for steel processing and properties.
Carbon's Influence on Iron's Properties
The presence of carbon as an interstitial solute dramatically alters the properties of iron, transforming it from relatively soft and ductile pure iron into strong and versatile steels. Let's examine the key influences:
Strengthening Mechanisms
Carbon atoms, when occupying interstitial sites, hinder the movement of dislocations – crystallographic defects that govern plastic deformation. This leads to several strengthening mechanisms:
-
Solid Solution Strengthening: The presence of carbon atoms within the iron lattice creates lattice distortions and stress fields. These distortions impede the motion of dislocations, thereby increasing the material's yield strength and hardness.
-
Interstitial Strengthening: Carbon atoms directly obstruct the movement of dislocations, requiring higher stresses for plastic deformation to occur.
The degree of strengthening is directly related to the carbon concentration. Higher carbon content generally leads to greater strength and hardness but often at the expense of ductility.
Influence on Phase Transformations
The interaction between carbon and iron is intricately linked to phase transformations, particularly the formation of cementite (Fe₃C). This compound is a crucial phase in steel, significantly influencing its microstructure and properties.
-
Eutectoid Transformation: The eutectoid transformation is a key phase transformation in iron-carbon alloys. At a specific carbon concentration (0.77 wt%), austenite (γ-iron) transforms into a mixture of ferrite (α-iron) and cementite (Fe₃C) upon cooling. This microstructure, known as pearlite, is characteristic of eutectoid steel and contributes to its high strength.
-
Hypo-eutectoid and Hyper-eutectoid Steels: Steels with less than 0.77 wt% carbon are called hypo-eutectoid steels, while those with more than 0.77 wt% are hyper-eutectoid steels. Their microstructures and properties vary depending on the carbon content and cooling rate, with variations in the amount and distribution of ferrite and cementite influencing their final properties.
Influence on Mechanical Properties
The carbon content in iron drastically affects its mechanical properties:
-
Yield Strength and Tensile Strength: Increasing carbon content significantly increases both yield and tensile strengths. This is primarily due to the solid solution and interstitial strengthening mechanisms already discussed.
-
Hardness: Higher carbon content translates to increased hardness, making the material more resistant to indentation and wear.
-
Ductility and Toughness: While carbon enhances strength and hardness, it often reduces ductility (ability to deform plastically before fracture) and toughness (resistance to fracture under impact). This trade-off between strength and ductility is a critical consideration in material selection.
-
Elastic Modulus: The elastic modulus, a measure of stiffness, is relatively less affected by moderate carbon additions, though subtle changes can occur due to lattice distortion.
Phase Diagrams and their Importance
Iron-carbon phase diagrams are crucial tools for understanding the relationship between temperature, carbon content, and the resulting phases. These diagrams predict the microstructure and, consequently, the mechanical properties of steel at various temperatures and carbon concentrations. Analyzing these diagrams allows metallurgists to control the heat treatment processes and achieve the desired properties.
The key regions in the iron-carbon phase diagram include:
-
Austenite Region: The region where γ-iron (FCC) is the stable phase, able to dissolve significant amounts of carbon.
-
Ferrite Region: The region where α-iron (BCC) is the stable phase, with low carbon solubility.
-
Cementite Region: The region where the iron carbide (Fe₃C) phase is present.
-
Eutectoid Point: The invariant point at 0.77 wt% carbon and 727°C, where austenite transforms to pearlite upon cooling.
Studying the phase diagram provides insights into heat treatment processes like annealing, normalizing, and quenching, which are used to modify the microstructure and achieve desired mechanical properties.
Applications of C-Fe Interstitial Solid Solutions
The versatile properties of carbon-iron interstitial solid solutions make them invaluable in countless applications:
-
Structural Steels: These steels, with varying carbon contents, are used extensively in construction, automotive, and shipbuilding industries due to their strength, weldability, and cost-effectiveness.
-
Tool Steels: High-carbon steels are used in the manufacturing of cutting tools due to their exceptional hardness and wear resistance.
-
Cast Irons: Cast irons contain higher carbon contents, along with other alloying elements, resulting in unique microstructures and properties that make them suitable for castings.
-
Specialty Steels: Various alloying elements are added to carbon steels to enhance specific properties like corrosion resistance (stainless steels), high-temperature strength, or magnetic properties.
Advanced Techniques for Characterizing C in Fe
Several advanced techniques are used to analyze the distribution and behavior of carbon in iron:
-
X-ray Diffraction (XRD): XRD helps determine the crystal structure and phase composition of the steel.
-
Transmission Electron Microscopy (TEM): TEM offers high-resolution imaging of the microstructure, revealing details about the distribution of carbon and other phases.
-
Auger Electron Spectroscopy (AES): AES is a surface-sensitive technique that provides compositional information on the surface layers, useful for analyzing carburization or decarburization processes.
-
Electron Probe Microanalysis (EPMA): EPMA is used for quantitative analysis of the elemental composition across different regions within the material.
These techniques are crucial in understanding the intricacies of interstitial solid solutions and optimizing material properties.
Future Research Directions
Ongoing research continues to refine our understanding of interstitial solid solutions of carbon in iron. Areas of active investigation include:
-
Advanced Alloying: Developing new alloying strategies to enhance specific properties, such as strength-to-weight ratio or corrosion resistance.
-
Computational Modeling: Utilizing advanced computational techniques like density functional theory (DFT) to simulate the atomic-level interactions between carbon and iron, aiding in the design of novel materials.
-
Advanced Characterization Techniques: Developing more sensitive and sophisticated characterization techniques to further understand the behavior of carbon within the iron matrix.
The study of interstitial solid solutions of carbon in iron is a dynamic field of research with far-reaching implications for materials science and engineering. Continued advancements in understanding and manipulating these materials promise to lead to new innovations in various industrial sectors. The fundamental relationship between carbon and iron remains a cornerstone of materials science, driving progress in the development of stronger, lighter, and more durable materials for diverse applications.
Latest Posts
Latest Posts
-
What Is 6 Quarts In Litres
May 19, 2025
-
How Many Inches In 110 Cm
May 19, 2025
-
How Many Days Are In 60 Hours
May 19, 2025
-
How Many Pounds Is 83 Kilos
May 19, 2025
-
53 Inches Is How Many Feet
May 19, 2025
Related Post
Thank you for visiting our website which covers about Interstitial Solid Solution C Solute In Fe . We hope the information provided has been useful to you. Feel free to contact us if you have any questions or need further assistance. See you next time and don't miss to bookmark.