In Order For A Process To Be Spontaneous
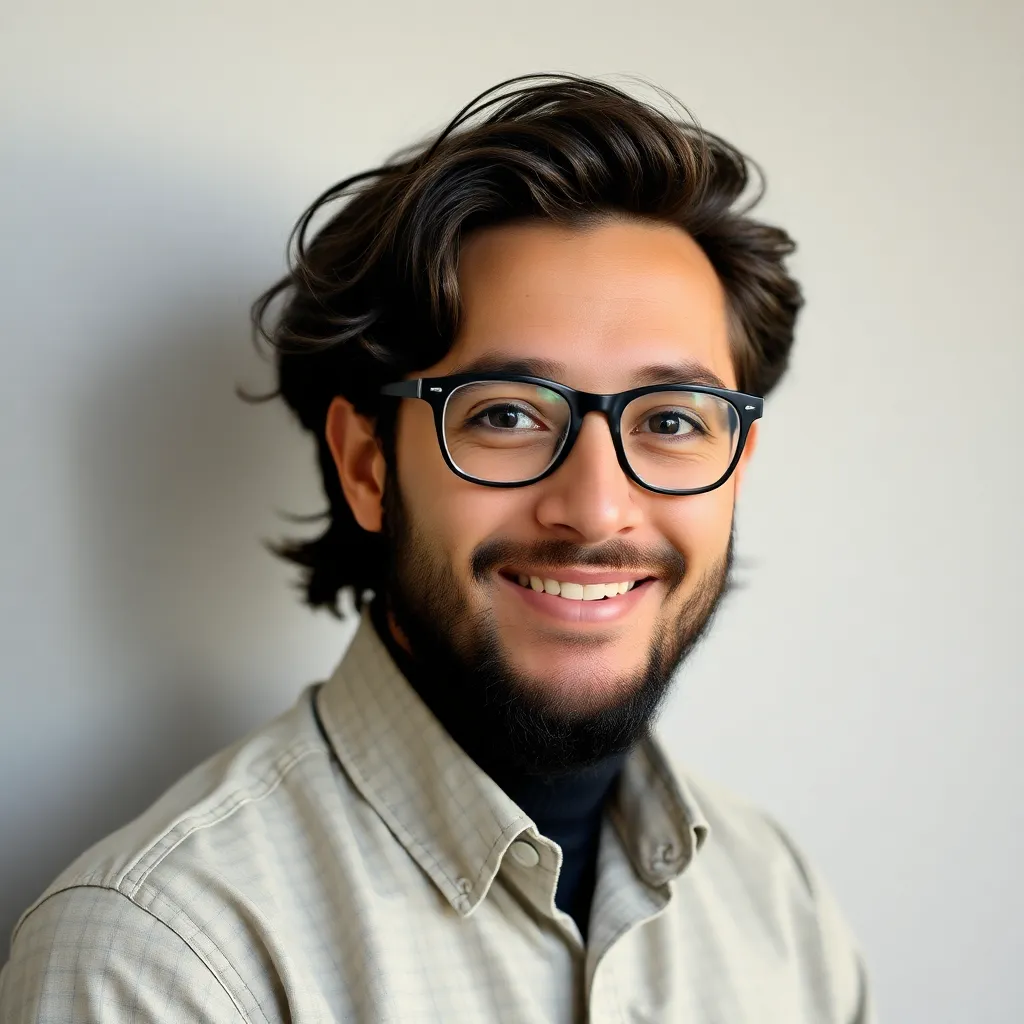
Holbox
May 10, 2025 · 6 min read
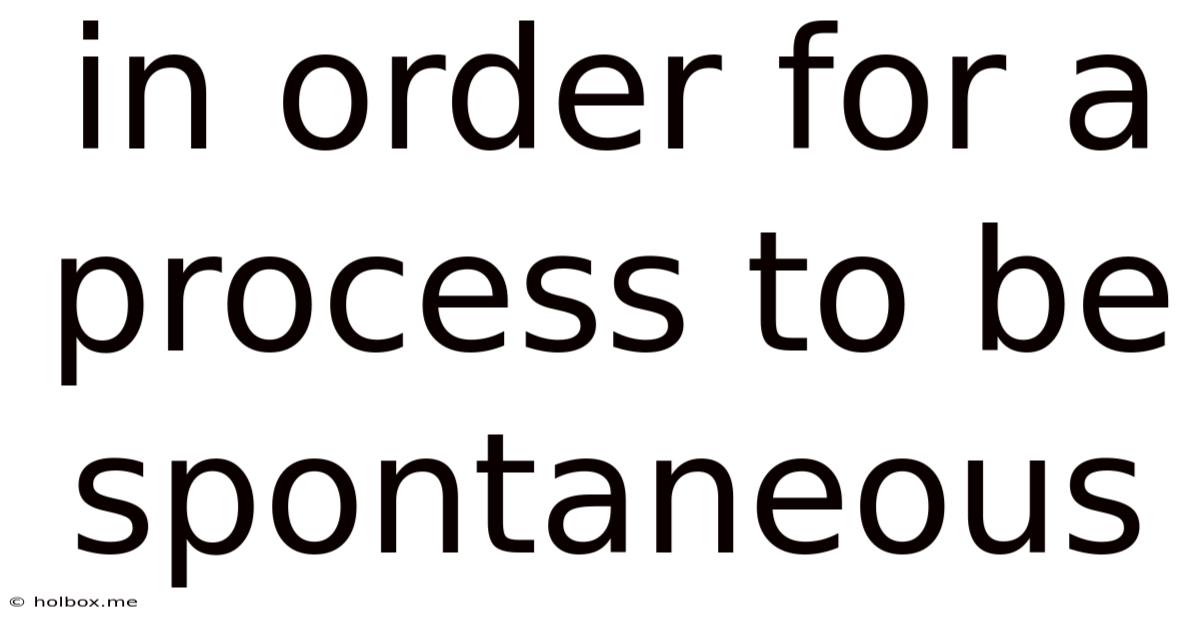
Table of Contents
- In Order For A Process To Be Spontaneous
- Table of Contents
- In Order for a Process to be Spontaneous: A Deep Dive into Thermodynamics and Entropy
- The Role of Entropy: Disorder's Reign
- Microscopic Perspective on Entropy
- Entropy Changes (ΔS): Predicting Spontaneity
- Enthalpy: The Energy Factor
- Gibbs Free Energy: The Decisive Factor
- Temperature's Influence on Spontaneity
- Non-Standard Conditions and the Equilibrium Constant
- Applications of Spontaneity Principles
- Factors Beyond Thermodynamics: Kinetics and Activation Energy
- Conclusion
- Latest Posts
- Related Post
In Order for a Process to be Spontaneous: A Deep Dive into Thermodynamics and Entropy
Spontaneity, in the context of thermodynamics, doesn't refer to a process happening quickly. Instead, it describes whether a process will occur naturally without external intervention. Understanding what makes a process spontaneous is crucial in chemistry, physics, and many other scientific fields. This article will explore the fundamental principles governing spontaneity, focusing on entropy, enthalpy, Gibbs Free Energy, and their interrelationships.
The Role of Entropy: Disorder's Reign
At the heart of spontaneity lies entropy (S), a measure of disorder or randomness in a system. The second law of thermodynamics states that the total entropy of an isolated system can only increase over time, or remain constant in ideal cases where the system is in a steady state or undergoing a reversible process. In simpler terms, nature tends towards chaos.
Microscopic Perspective on Entropy
Imagine a perfectly ordered deck of cards. The probability of finding it in this ordered state is incredibly low. Shuffling the deck increases the disorder; the probability of finding any specific arrangement becomes much higher, reflecting the increase in entropy. Similarly, in a chemical system, a highly ordered arrangement of molecules (e.g., a pure crystal) has low entropy, while a more disordered arrangement (e.g., a gas) has high entropy.
Entropy Changes (ΔS): Predicting Spontaneity
The change in entropy (ΔS) for a process indicates the change in disorder. A positive ΔS (ΔS > 0) signifies an increase in disorder, favoring spontaneity. Conversely, a negative ΔS (ΔS < 0) indicates a decrease in disorder, hindering spontaneity.
-
Examples of Processes with Positive ΔS:
- Melting of a solid: The molecules transition from a highly ordered state to a more disordered liquid state.
- Boiling of a liquid: The molecules become even more disordered in the gaseous phase.
- Dissolving of a solid in a liquid: The solute particles spread out, increasing the overall disorder.
- Expansion of a gas: The gas molecules occupy a larger volume, leading to increased disorder.
-
Examples of Processes with Negative ΔS:
- Freezing of a liquid: The molecules become more ordered in the solid state.
- Condensation of a gas: The molecules become less disordered in the liquid state.
- Formation of a precipitate: Ions combine to form a solid, reducing disorder.
Enthalpy: The Energy Factor
While entropy points towards disorder, enthalpy (H) reflects the heat content of a system at constant pressure. Enthalpy changes (ΔH) during a process represent the heat absorbed or released.
-
Exothermic Processes (ΔH < 0): These processes release heat to the surroundings. The system's energy decreases, and heat is transferred to the environment, often contributing to spontaneity. Think of combustion reactions—they are exothermic and usually spontaneous.
-
Endothermic Processes (ΔH > 0): These processes absorb heat from the surroundings. The system's energy increases. Endothermic reactions are less likely to be spontaneous unless driven by a large entropy increase. Melting ice is an example; it requires heat input and has a positive ΔH.
Gibbs Free Energy: The Decisive Factor
Combining entropy and enthalpy, Gibbs Free Energy (G) provides a more comprehensive measure of spontaneity. Defined as G = H – TS (where T is the absolute temperature), the change in Gibbs Free Energy (ΔG) determines the spontaneity of a process at constant temperature and pressure.
-
ΔG < 0 (Negative): The process is spontaneous under the given conditions. The decrease in Gibbs Free Energy represents a more favorable, lower-energy state.
-
ΔG > 0 (Positive): The process is non-spontaneous under the given conditions. External energy input is required for it to occur.
-
ΔG = 0 (Zero): The process is at equilibrium. There is no net change in the system's state.
The relationship between ΔH, ΔS, and ΔG is summarized as follows:
ΔH | ΔS | ΔG | Spontaneity |
---|---|---|---|
< 0 | > 0 | < 0 | Always spontaneous |
< 0 | < 0 | Depends on T | Spontaneous at low temperatures (ΔH < TΔS) |
> 0 | > 0 | Depends on T | Spontaneous at high temperatures (TΔS > ΔH) |
> 0 | < 0 | > 0 | Never spontaneous |
Temperature's Influence on Spontaneity
Temperature plays a critical role in determining spontaneity, especially when ΔH and ΔS have opposite signs.
-
Low Temperatures: At lower temperatures, the TΔS term in the Gibbs Free Energy equation is small. Therefore, the enthalpy term (ΔH) dominates. Exothermic processes (ΔH < 0) are more likely to be spontaneous.
-
High Temperatures: At higher temperatures, the TΔS term becomes significant. Processes with a large positive entropy change (ΔS > 0) can become spontaneous, even if they are endothermic (ΔH > 0). This is because the increase in disorder outweighs the energy cost.
Non-Standard Conditions and the Equilibrium Constant
The discussions so far have primarily focused on standard conditions. However, spontaneity can vary under non-standard conditions. The equilibrium constant (K) helps predict the spontaneity of a reaction under non-standard conditions. A larger K indicates a more spontaneous reaction. The relationship between ΔG and K is given by:
ΔG = -RTlnK
where R is the gas constant and T is the temperature in Kelvin.
Applications of Spontaneity Principles
Understanding spontaneity has profound implications across various fields:
-
Chemistry: Predicting the feasibility of chemical reactions, designing efficient reaction pathways, and understanding equilibrium states.
-
Material Science: Designing new materials with desired properties by controlling the spontaneity of phase transitions and chemical reactions.
-
Biology: Understanding metabolic processes, protein folding, and other biological phenomena.
-
Environmental Science: Studying the spontaneity of environmental processes, such as pollution dispersion and geochemical transformations.
Factors Beyond Thermodynamics: Kinetics and Activation Energy
It is crucial to remember that spontaneity only indicates the likelihood of a process occurring; it doesn't determine how fast it will happen. A spontaneous reaction might be incredibly slow if the activation energy (the energy required to initiate the reaction) is very high. This is where kinetics, the study of reaction rates, comes into play. A spontaneous reaction with a high activation energy will require a catalyst to proceed at an appreciable rate.
For instance, the combustion of methane (natural gas) is highly spontaneous, releasing a substantial amount of energy. However, a methane-air mixture will not spontaneously combust without an ignition source to provide the necessary activation energy.
Conclusion
Spontaneity is a fundamental concept in thermodynamics, governed by the interplay of enthalpy, entropy, and temperature. Gibbs Free Energy provides a powerful tool for predicting spontaneity under constant temperature and pressure conditions. While spontaneity indicates the thermodynamic feasibility of a process, the rate at which it proceeds is determined by kinetic factors. A deep understanding of these principles is essential across numerous scientific and engineering disciplines. By carefully considering enthalpy, entropy, and temperature, we can predict and manipulate the spontaneity of processes to achieve desired outcomes in various applications. Further exploration of advanced thermodynamic concepts, such as non-standard conditions and coupled reactions, will provide a more complete understanding of this vital field.
Latest Posts
Related Post
Thank you for visiting our website which covers about In Order For A Process To Be Spontaneous . We hope the information provided has been useful to you. Feel free to contact us if you have any questions or need further assistance. See you next time and don't miss to bookmark.