Graded Potentials Result From The Opening Of
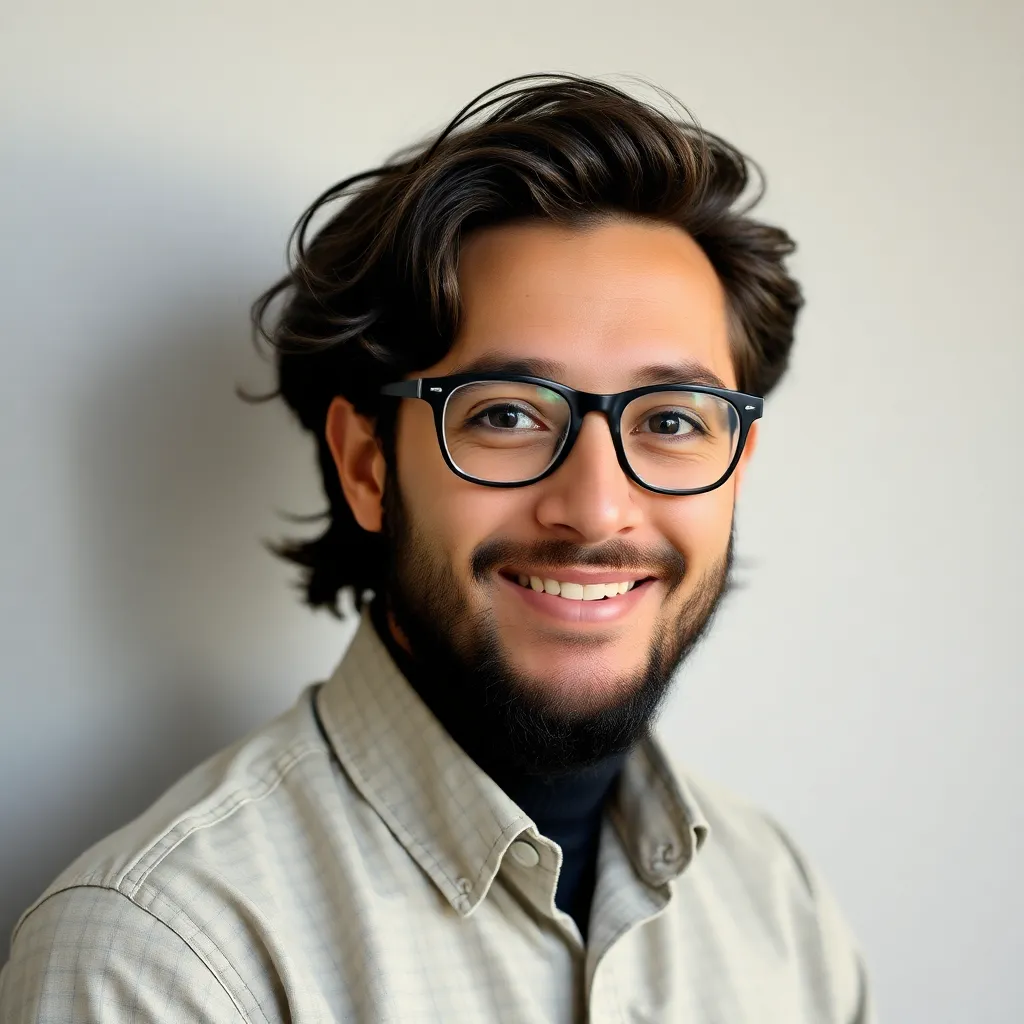
Holbox
May 11, 2025 · 7 min read
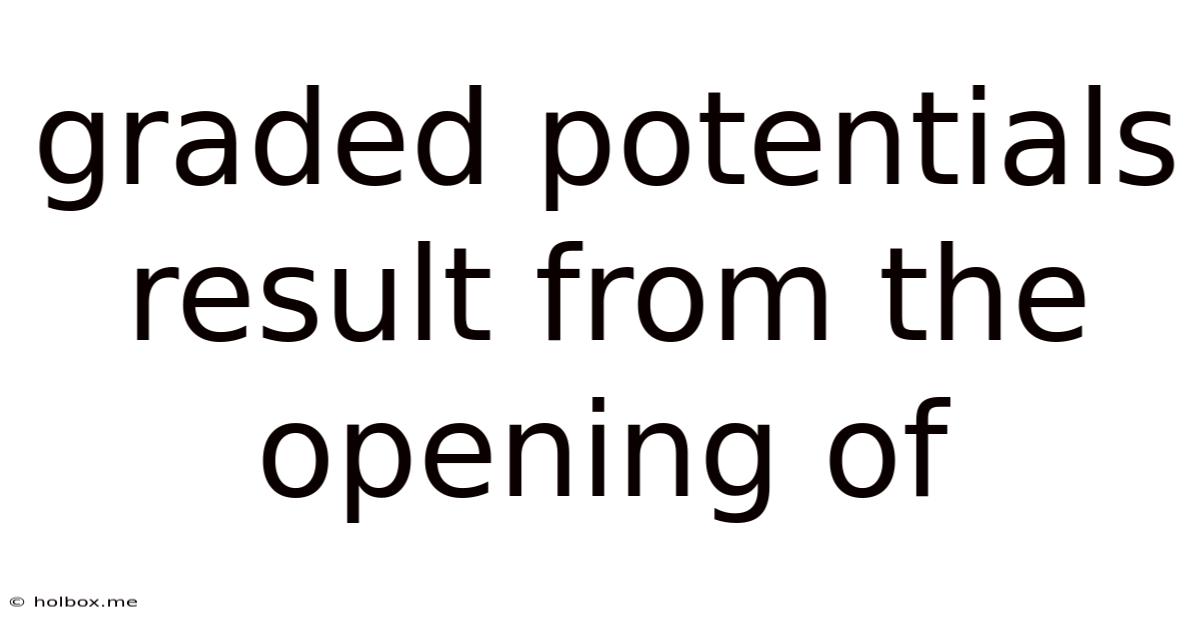
Table of Contents
- Graded Potentials Result From The Opening Of
- Table of Contents
- Graded Potentials: Resulting from the Opening of Ligand-Gated and Mechanically-Gated Ion Channels
- Understanding Ion Channels and Membrane Potential
- The Role of Ligand-Gated Ion Channels in Graded Potentials
- The Role of Mechanically-Gated Ion Channels in Graded Potentials
- Characteristics of Graded Potentials
- Graded Potentials and Action Potential Initiation
- Physiological Significance of Graded Potentials
- Conclusion
- Latest Posts
- Related Post
Graded Potentials: Resulting from the Opening of Ligand-Gated and Mechanically-Gated Ion Channels
Graded potentials are transient changes in the membrane potential of a neuron or other excitable cell. Unlike action potentials, which are all-or-none events, graded potentials are variable in amplitude and duration. Their magnitude is directly proportional to the strength of the stimulus, and they can summate (add together) over time and space. Crucially, graded potentials are the result of the opening of specific ion channels – primarily ligand-gated and mechanically-gated channels. This article will delve deep into the mechanisms underlying graded potentials, exploring their generation, characteristics, and physiological significance.
Understanding Ion Channels and Membrane Potential
Before diving into graded potentials, it's essential to grasp the fundamental principles of ion channels and membrane potential. The cell membrane, a lipid bilayer, acts as a barrier to the free movement of ions. However, specialized protein channels embedded within this membrane allow selective passage of specific ions, like sodium (Na⁺), potassium (K⁺), calcium (Ca²⁺), and chloride (Cl⁻).
The resting membrane potential, typically around -70 mV in neurons, is established by the unequal distribution of these ions across the membrane and the selective permeability of the membrane to these ions. This unequal distribution is maintained by the sodium-potassium pump, which actively transports Na⁺ out of the cell and K⁺ into the cell against their concentration gradients. At rest, the membrane is more permeable to K⁺ than Na⁺, leading to a negative intracellular charge.
The Role of Ligand-Gated Ion Channels in Graded Potentials
Ligand-gated ion channels, also known as chemically-gated ion channels, are a crucial type of ion channel responsible for initiating graded potentials. These channels open or close in response to the binding of a specific neurotransmitter or ligand to their receptor site. The neurotransmitter, released from a presynaptic neuron, diffuses across the synaptic cleft and binds to the receptors on the postsynaptic neuron's membrane, triggering the opening of the ligand-gated ion channels.
Examples of Ligand-Gated Ion Channels and their Effects:
-
Nicotinic acetylcholine receptors: These receptors, found at the neuromuscular junction and in the autonomic nervous system, are permeable to both Na⁺ and K⁺. Upon acetylcholine binding, they open, leading to a depolarization (less negative membrane potential) due to the greater influx of Na⁺ compared to the efflux of K⁺. This depolarization is a type of excitatory postsynaptic potential (EPSP).
-
GABA<sub>A</sub> receptors: These receptors bind the neurotransmitter GABA (gamma-aminobutyric acid), a major inhibitory neurotransmitter in the central nervous system. When activated, they allow Cl⁻ influx into the neuron, leading to hyperpolarization (more negative membrane potential). This hyperpolarization is an inhibitory postsynaptic potential (IPSP).
-
Glutamate receptors (AMPA and NMDA): Glutamate, the primary excitatory neurotransmitter in the brain, binds to AMPA and NMDA receptors, causing depolarization. AMPA receptors are primarily permeable to Na⁺, while NMDA receptors allow both Na⁺ and Ca²⁺ to pass. The influx of these positive ions generates EPSPs.
The opening of ligand-gated ion channels leads to a localized change in membrane potential. The amplitude of this change is directly proportional to the amount of neurotransmitter released and the number of receptors activated. If multiple ligand-gated ion channels open simultaneously or in rapid succession, the individual graded potentials can summate, leading to a larger overall change in membrane potential.
The Role of Mechanically-Gated Ion Channels in Graded Potentials
Mechanically-gated ion channels are another critical player in generating graded potentials. These channels open or close in response to mechanical stimuli, such as stretch, pressure, or vibration. They are found in various locations throughout the body, including the skin, hair cells of the inner ear, and baroreceptors in blood vessels.
Examples of Mechanically-Gated Ion Channels and their Effects:
-
Hair cells in the inner ear: These cells contain mechanically-gated ion channels that open when the stereocilia (hair-like projections) are deflected by sound vibrations. This opening leads to depolarization and the initiation of action potentials that transmit auditory information to the brain.
-
Baroreceptors in blood vessels: Baroreceptors are specialized sensory neurons that detect changes in blood pressure. Stretching of the blood vessel wall opens mechanically-gated ion channels in the baroreceptors, leading to depolarization and an increase in the firing rate of action potentials. This information is transmitted to the brain and helps regulate blood pressure.
-
Tactile receptors in the skin: Mechanically-gated ion channels in tactile receptors respond to touch, pressure, and vibration. These receptors initiate graded potentials that are transmitted to the brain, allowing us to perceive different tactile sensations.
Similar to ligand-gated channels, the opening of mechanically-gated ion channels generates a localized change in membrane potential. The amplitude of this change is directly related to the intensity of the mechanical stimulus.
Characteristics of Graded Potentials
Graded potentials differ significantly from action potentials in several key characteristics:
-
Amplitude: Graded potentials have variable amplitudes; their size is directly proportional to the strength of the stimulus. A stronger stimulus opens more ion channels, leading to a larger change in membrane potential. In contrast, action potentials are all-or-none events; they either occur with a consistent amplitude or not at all.
-
Duration: Graded potentials have variable durations; they decay over time if the stimulus is removed. The duration is influenced by factors such as the time the channels remain open and the rate of ion diffusion. Action potentials have a relatively fixed duration.
-
Summation: Graded potentials can summate, both temporally (multiple stimuli in rapid succession) and spatially (multiple stimuli at different locations). This summation can lead to a larger overall change in membrane potential, which can potentially trigger an action potential. Action potentials are incapable of summation.
-
Decremental Conduction: Graded potentials exhibit decremental conduction, meaning their amplitude decreases as they travel away from the point of stimulation. This is due to leakage of ions across the membrane. Action potentials, on the other hand, exhibit non-decremental conduction, maintaining their amplitude as they propagate down the axon.
-
Location: Graded potentials occur in the dendrites and cell body of a neuron, while action potentials are generated at the axon hillock and propagate down the axon.
Graded Potentials and Action Potential Initiation
Graded potentials, whether EPSPs or IPSPs, play a crucial role in determining whether an action potential is generated. The sum of all graded potentials arriving at the axon hillock (the trigger zone) determines the net membrane potential. If the net membrane potential reaches the threshold potential (typically around -55 mV), voltage-gated sodium channels open, initiating an action potential. If the net membrane potential fails to reach the threshold, no action potential is generated.
Therefore, graded potentials act as a form of signal integration, summing both excitatory and inhibitory inputs to determine the neuron's output. This allows for complex processing of information within the nervous system.
Physiological Significance of Graded Potentials
Graded potentials are vital for a wide range of physiological processes:
-
Synaptic Transmission: They form the basis of communication between neurons at synapses.
-
Sensory Transduction: They convert sensory stimuli (light, sound, touch, pressure, temperature) into electrical signals that the nervous system can interpret.
-
Muscle Contraction: They trigger muscle contractions in response to nerve impulses at the neuromuscular junction.
-
Hormone Secretion: They regulate the release of hormones from endocrine glands.
-
Control of Heart Rate: They help control the rate of heartbeat via baroreceptor reflexes.
Conclusion
Graded potentials are essential signaling mechanisms in excitable cells. Their properties, arising from the opening of ligand-gated and mechanically-gated ion channels, allow for precise control of membrane potential and information processing. The ability of graded potentials to summate and influence the initiation of action potentials underlies their critical role in neuronal communication, sensory transduction, and countless other physiological functions. Further research into the intricacies of ion channels and their interactions continues to expand our understanding of these crucial cellular processes and their impact on human health and disease. Understanding these mechanisms is vital for comprehending how the nervous system functions and for developing potential therapies for neurological disorders. The complex interplay of graded potentials and action potentials demonstrates the remarkable sophistication of cellular communication and its critical role in the overall function of the human body.
Latest Posts
Related Post
Thank you for visiting our website which covers about Graded Potentials Result From The Opening Of . We hope the information provided has been useful to you. Feel free to contact us if you have any questions or need further assistance. See you next time and don't miss to bookmark.