Evaluate The Representation Of Ionic Bonding
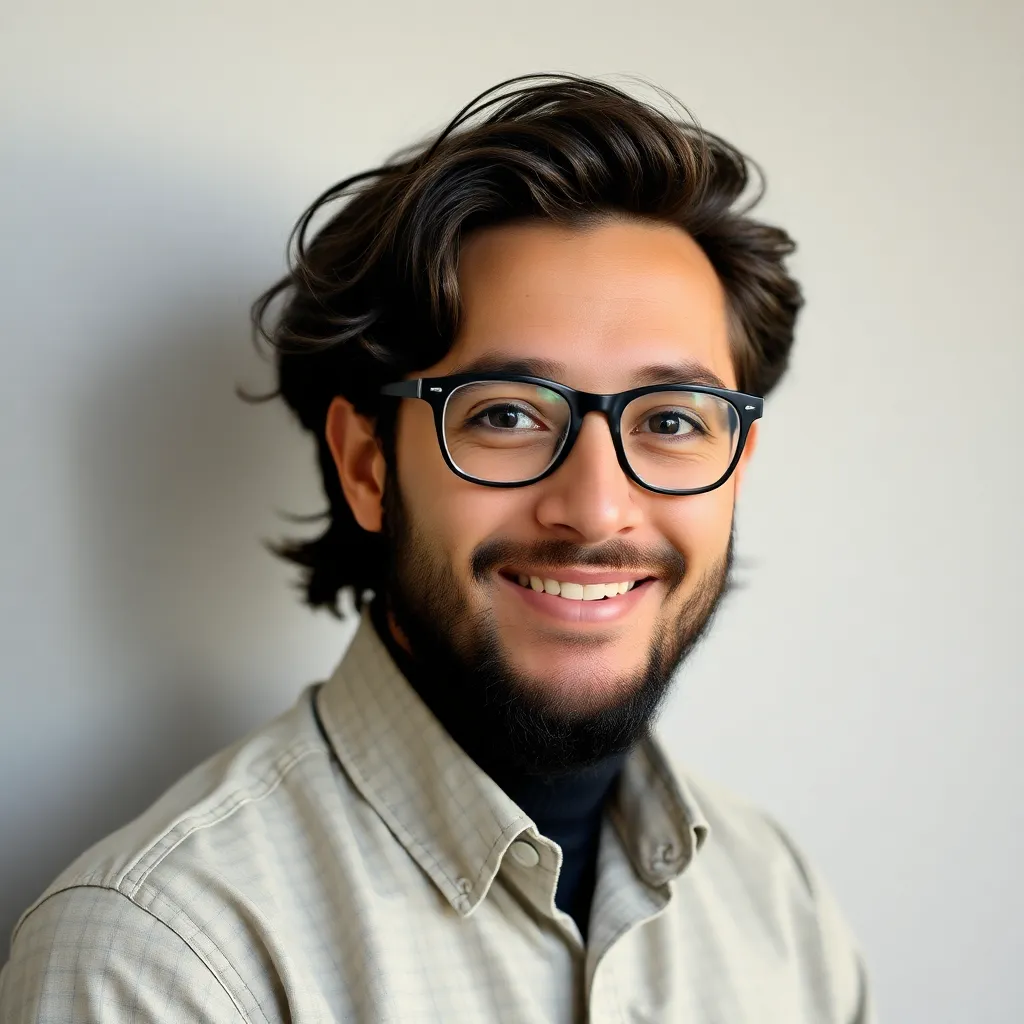
Holbox
May 11, 2025 · 6 min read
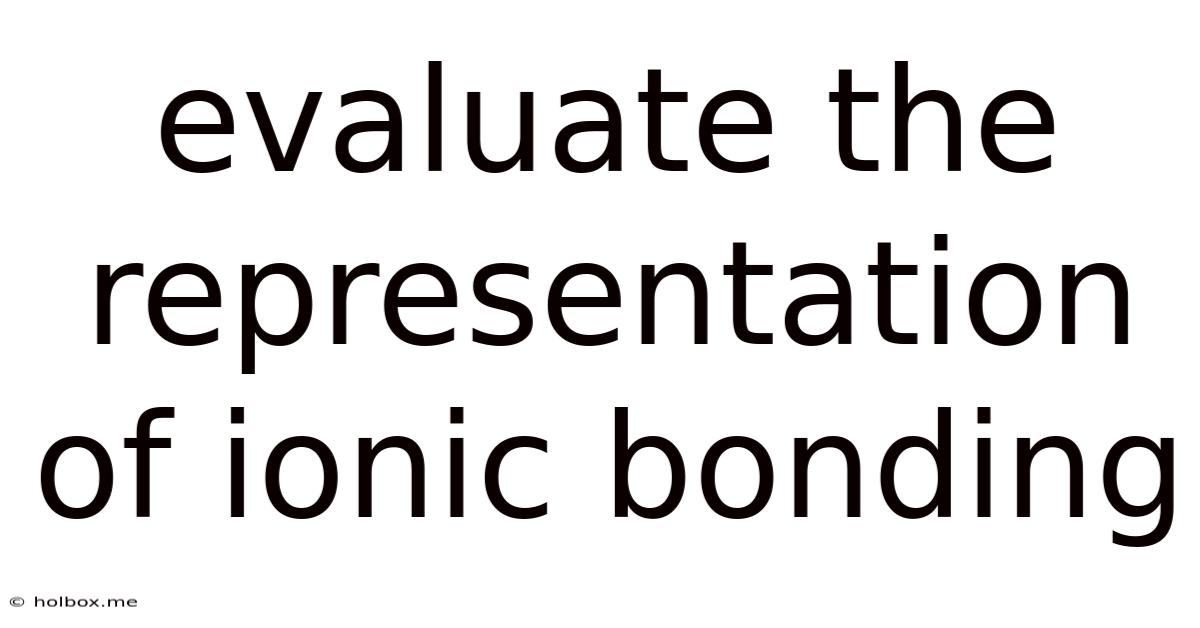
Table of Contents
- Evaluate The Representation Of Ionic Bonding
- Table of Contents
- Evaluating the Representation of Ionic Bonding
- The Classical Model: Strengths and Limitations
- Beyond the Classical Model: More Advanced Representations
- Born-Haber Cycle
- Electrostatic Potential Maps
- Molecular Orbital Theory
- Crystal Field Theory (for transition metal complexes)
- The Importance of Considering Covalent Character
- Limitations of Visual Representations
- Conclusion: Towards a More Holistic Understanding
- Latest Posts
- Latest Posts
- Related Post
Evaluating the Representation of Ionic Bonding
Ionic bonding, a fundamental concept in chemistry, describes the electrostatic attraction between oppositely charged ions. While seemingly straightforward, accurately representing ionic bonding requires careful consideration of various factors, encompassing both its theoretical underpinnings and practical limitations. This article delves into a comprehensive evaluation of how ionic bonding is represented, highlighting its strengths and weaknesses across different models and representations.
The Classical Model: Strengths and Limitations
The most basic representation of ionic bonding involves the transfer of electrons from a metal atom (low electronegativity) to a non-metal atom (high electronegativity), forming positively charged cations and negatively charged anions. This is often depicted using Lewis dot structures, illustrating the valence electrons and their transfer. For example, the formation of sodium chloride (NaCl) is shown with sodium losing one electron to achieve a stable octet, becoming Na+, and chlorine gaining one electron to achieve a stable octet, becoming Cl-.
Strengths of the Classical Model:
- Simplicity and Conceptual Clarity: This model provides a simple and intuitive understanding of the fundamental process involved in ionic bond formation. It's easily grasped by beginners in chemistry and serves as a strong foundation for further learning.
- Explains Basic Properties: The classical model effectively explains some key properties of ionic compounds, such as their high melting and boiling points (due to strong electrostatic forces) and their ability to conduct electricity when molten or dissolved in water (due to the presence of mobile ions).
- Predictive Power (to an extent): The model allows for the prediction of the charges of ions based on their position in the periodic table and can be used to predict the formulas of simple ionic compounds.
Limitations of the Classical Model:
- Oversimplification of Electron Transfer: The complete transfer of electrons is an oversimplification. In reality, there's always some degree of electron sharing or covalent character, particularly in compounds involving highly charged ions or ions with similar electronegativities. This is especially true for compounds that are considered "ionic" but exhibit significant covalent character, like many transition metal compounds.
- Ignoring the Role of Lattice Energy: The classical model neglects the crucial role of lattice energy – the energy released when ions come together to form a crystalline lattice. The stability of an ionic compound isn't solely dependent on the individual ions achieving noble gas configurations but also on the overall lattice energy, which dictates the strength of the ionic bond.
- Failure to Account for Polarizability: The classical model doesn't account for the polarizability of ions. Larger ions are more polarizable than smaller ions, meaning their electron clouds are more easily distorted by the electric field of neighboring ions. This polarizability influences the strength of the ionic bond and other properties of the compound.
- Incomplete Description of Crystal Structure: The classical model doesn't explain the intricate three-dimensional crystal structures adopted by ionic compounds. Factors like ion size and charge ratios determine the specific crystal structure, which significantly influences the physical and chemical properties of the compound.
Beyond the Classical Model: More Advanced Representations
To overcome the limitations of the classical model, more sophisticated representations are employed.
Born-Haber Cycle
The Born-Haber cycle is a thermodynamic approach to understanding ionic bond formation. It accounts for various energy changes associated with the formation of an ionic compound from its constituent elements, including ionization energy, electron affinity, lattice energy, and sublimation energy. By applying Hess's law, the Born-Haber cycle allows for the calculation of lattice energy, a crucial parameter in assessing the stability of an ionic compound. This cycle provides a more quantitative understanding than the simple electron transfer model.
Electrostatic Potential Maps
Electrostatic potential maps (EPMs) are visual representations of the charge distribution in a molecule or ion. These maps provide a valuable tool for understanding the distribution of electron density and the regions of positive and negative charge within an ionic compound. They highlight the polarization of ions and the unequal sharing of electrons, showcasing the limitations of the purely ionic model. They better represent the reality of ionic bonding with often-present covalent character.
Molecular Orbital Theory
While primarily used for covalent bonding, molecular orbital theory (MOT) can be adapted to describe ionic bonding. It considers the interaction of atomic orbitals to form bonding and antibonding molecular orbitals. In ionic compounds, the electron transfer can be viewed as a significant difference in the occupancy of these molecular orbitals.
Crystal Field Theory (for transition metal complexes)
For transition metal complexes which can have significant ionic character, Crystal Field Theory (CFT) offers a deeper understanding. It models the interaction between the metal ion and the surrounding ligands (ions or molecules) using an electrostatic model. CFT explains the splitting of d-orbitals in the presence of ligands, and hence, explains the color, magnetism, and other properties of these compounds. This demonstrates the complexity that even "ionic" compounds can exhibit.
The Importance of Considering Covalent Character
A crucial aspect often overlooked in simplified representations of ionic bonding is the existence of covalent character. Even in compounds considered highly ionic, some degree of electron sharing occurs. This arises from the polarization of ions, where the electron cloud of one ion is distorted by the electric field of the other. The greater the charge density and the smaller the ion, the greater the polarization and the greater the covalent character. This covalent character influences the physical and chemical properties of the ionic compound.
Factors Influencing Covalent Character:
- Charge Density of Ions: Highly charged ions exhibit greater polarization and thus greater covalent character.
- Size of Ions: Smaller ions are more polarizing and lead to greater covalent character.
- Electronegativity Difference: While a large electronegativity difference generally suggests ionic bonding, a small difference can still result in some degree of covalent character.
The extent of covalent character can be evaluated using various parameters, including the dipole moment, spectroscopic data, and theoretical calculations.
Limitations of Visual Representations
Representations of ionic bonding, even sophisticated ones, have limitations:
- Static Nature: Many visual representations are static snapshots, failing to capture the dynamic nature of ionic interactions within a crystal lattice. Ions are constantly vibrating and their interactions are influenced by temperature and other factors.
- Scale Limitations: It's impossible to perfectly represent the relative sizes and distances between ions in a crystal lattice in a visually accessible way.
- Simplification of Complex Interactions: Representations often simplify complex interactions, such as van der Waals forces and polarization effects, which play a significant role in influencing the properties of ionic compounds.
Conclusion: Towards a More Holistic Understanding
The representation of ionic bonding evolves with advances in our understanding of chemical interactions. While the classical model provides a useful starting point, a comprehensive understanding necessitates the incorporation of more sophisticated models like the Born-Haber cycle, electrostatic potential maps, molecular orbital theory, and crystal field theory (where applicable). Acknowledging and quantifying the presence of covalent character is also essential for a complete and accurate picture. The ongoing development and refinement of computational techniques offer further opportunities to enhance our visualization and understanding of ionic bonding, bridging the gap between simplified models and the complex reality of interatomic interactions. Future research will likely focus on bridging the traditional dichotomy between ionic and covalent bonding to provide a more unified and comprehensive framework. Ultimately, the "best" representation depends on the level of detail and specific properties being investigated.
Latest Posts
Latest Posts
-
156 Ml Is How Many Ounces
May 18, 2025
-
4500 G In Lbs And Oz
May 18, 2025
-
28 Oz Is How Many Ml
May 18, 2025
-
How Many Days Is 2000 Hours
May 18, 2025
-
How Many Cups In 6 Liters
May 18, 2025
Related Post
Thank you for visiting our website which covers about Evaluate The Representation Of Ionic Bonding . We hope the information provided has been useful to you. Feel free to contact us if you have any questions or need further assistance. See you next time and don't miss to bookmark.