Essentials Of Radiographic Physics And Imaging
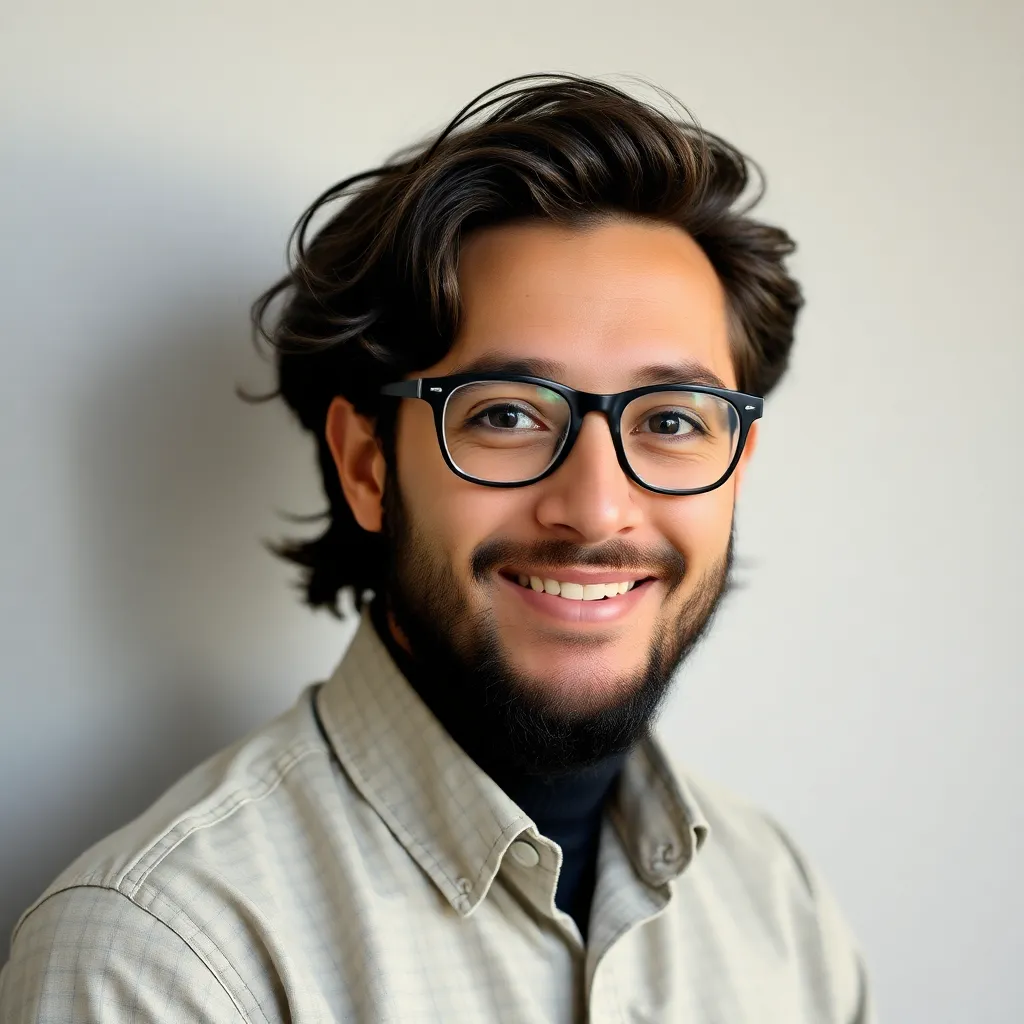
Holbox
May 13, 2025 · 6 min read
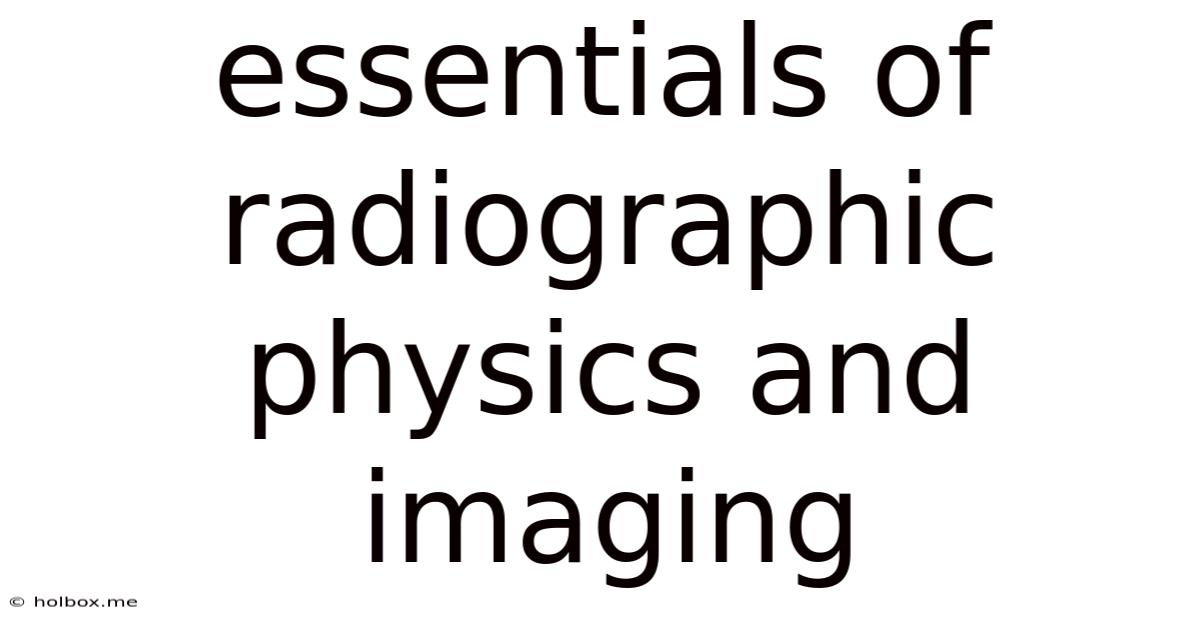
Table of Contents
- Essentials Of Radiographic Physics And Imaging
- Table of Contents
- Essentials of Radiographic Physics and Imaging
- X-Ray Production: The Heart of Radiography
- Thermionic Emission: The Electron Source
- Acceleration and Impact: Generating X-Rays
- Bremsstrahlung Radiation: The Majority of X-Rays
- Characteristic Radiation: Specific Energy Levels
- X-Ray Beam Properties: Understanding the Output
- X-Ray Interactions with Matter: The Basis of Image Formation
- Photoelectric Absorption: Complete Absorption
- Compton Scattering: Partial Absorption
- Coherent Scattering: Negligible in Diagnostic Imaging
- Image Formation and Detectors: Converting X-Rays into Images
- Image Receptors: Capturing the X-Ray Signal
- Image Processing and Display: Enhancing Visual Information
- Signal Processing: Noise Reduction and Enhancement
- Image Display: Optimizing Visualization
- Radiation Protection: Minimizing Patient Dose
- ALARA Principle: As Low As Reasonably Achievable
- Optimization Techniques: Balancing Image Quality and Dose
- Conclusion: A Synergistic Blend of Physics and Imaging
- Latest Posts
- Latest Posts
- Related Post
Essentials of Radiographic Physics and Imaging
Radiographic imaging, a cornerstone of modern medical diagnostics, relies heavily on the principles of physics. Understanding these principles is crucial for radiographers to produce high-quality images, optimize radiation protection, and ensure patient safety. This article delves into the essentials of radiographic physics and imaging, covering key concepts from x-ray production to image formation and interpretation.
X-Ray Production: The Heart of Radiography
At the heart of radiographic imaging lies the x-ray tube, a sophisticated device designed to generate x-rays. This process hinges on the interaction between electrons and a target material, typically tungsten.
Thermionic Emission: The Electron Source
The process begins with thermionic emission. A filament, heated by an electric current, releases electrons through a process called thermionic emission. The hotter the filament, the more electrons are emitted. This electron cloud is crucial for the subsequent stages of x-ray production.
Acceleration and Impact: Generating X-Rays
These released electrons are then accelerated towards a target anode (usually tungsten) by a high voltage potential difference. The kinetic energy of these high-speed electrons is converted into x-rays upon impact with the target. This conversion is not perfectly efficient; much of the energy is lost as heat. This is why x-ray tubes require sophisticated cooling mechanisms.
Bremsstrahlung Radiation: The Majority of X-Rays
The majority of x-rays produced are bremsstrahlung radiation, also known as braking radiation. This occurs when the high-speed electrons are decelerated by the strong electric field of the tungsten atoms in the anode. This deceleration causes the electrons to lose energy, which is emitted as x-rays. The energy of these x-rays varies depending on the degree of deceleration.
Characteristic Radiation: Specific Energy Levels
A smaller portion of the x-rays produced are characteristic radiation. This type of radiation is generated when the incoming high-speed electron knocks an inner-shell electron out of a tungsten atom. An outer-shell electron then fills this vacancy, releasing energy in the form of an x-ray photon with a specific energy level, characteristic of the target material.
X-Ray Beam Properties: Understanding the Output
The x-ray beam emerging from the tube is not monochromatic; it comprises a spectrum of x-ray energies. This spectrum is influenced by several factors, including the kilovoltage peak (kVp), which determines the maximum energy of the x-rays, and the milliamperage (mA), which determines the number of electrons produced and hence the intensity of the beam. Understanding this energy spectrum is critical in optimizing image quality and minimizing patient dose.
X-Ray Interactions with Matter: The Basis of Image Formation
Once the x-ray beam exits the tube, it interacts with the tissues and structures within the patient's body. These interactions are crucial for image formation. The primary interactions are:
Photoelectric Absorption: Complete Absorption
Photoelectric absorption is a dominant interaction at lower x-ray energies. In this process, the x-ray photon interacts with an inner-shell electron, transferring all its energy to the electron and ejecting it. The photon is completely absorbed. The ejected electron then interacts with surrounding atoms, leading to further ionization and energy deposition within the tissue. The probability of photoelectric absorption is highly dependent on the atomic number (Z) of the tissue and the energy of the x-ray photon. Higher Z materials, such as bone, absorb more x-rays than lower Z materials like soft tissue.
Compton Scattering: Partial Absorption
Compton scattering is a significant interaction at higher x-ray energies. In this process, the x-ray photon interacts with an outer-shell electron, transferring only part of its energy to the electron. The photon is scattered in a new direction with reduced energy. Compton scattering contributes significantly to image degradation (scatter radiation) and increases patient dose.
Coherent Scattering: Negligible in Diagnostic Imaging
Coherent scattering, also known as Rayleigh scattering, involves the elastic scattering of the x-ray photon with little or no energy loss. It is relatively unimportant in diagnostic radiography because it contributes minimally to image formation and patient dose.
Image Formation and Detectors: Converting X-Rays into Images
The differential absorption of x-rays by different tissues forms the basis of radiographic imaging. Areas of high attenuation (e.g., bone) appear brighter on the image (radiopaque), while areas of low attenuation (e.g., air) appear darker (radiolucent).
Image Receptors: Capturing the X-Ray Signal
Modern radiography uses various image receptors to capture the attenuated x-ray beam:
-
Film-Screen Systems: Historically dominant, these systems use intensifying screens to convert x-rays into visible light, which then exposes photographic film. These systems are gradually being replaced by digital detectors due to their limitations in terms of image quality and efficiency.
-
Digital Radiography (DR): DR systems use solid-state detectors (e.g., flat-panel detectors) to directly convert x-rays into digital signals. These systems offer numerous advantages over film-screen systems, including improved image quality, faster image acquisition, and the ability for post-processing manipulation.
-
Computed Radiography (CR): CR systems utilize photostimulable phosphor plates to capture the x-ray image. These plates are then scanned by a reader to produce a digital image. CR offers a transitionary step from film-screen to direct digital systems, providing some of the benefits of digital imaging without requiring a complete replacement of equipment.
Image Processing and Display: Enhancing Visual Information
Once the x-ray signal is captured by the detector, it is processed and displayed as a digital image. This involves several steps:
Signal Processing: Noise Reduction and Enhancement
Signal processing techniques are employed to enhance image quality. This includes noise reduction algorithms, which reduce the random variations in the image signal, and various contrast enhancement methods, which improve the visibility of subtle details.
Image Display: Optimizing Visualization
The processed image is then displayed on a monitor for interpretation by a radiologist. The monitor's characteristics (e.g., brightness, contrast, resolution) play a vital role in the accuracy of image interpretation. Understanding the display characteristics is essential for optimal visualization.
Radiation Protection: Minimizing Patient Dose
Radiation protection is a paramount concern in radiographic imaging. Minimizing patient dose without compromising image quality is a key objective. This is achieved through several strategies:
ALARA Principle: As Low As Reasonably Achievable
The ALARA principle (As Low As Reasonably Achievable) guides all radiation protection practices. This principle dictates that all exposure to ionizing radiation should be kept as low as reasonably achievable, taking into account social and economic factors.
Optimization Techniques: Balancing Image Quality and Dose
Various techniques are employed to optimize radiation protection:
-
Collimation: Restricting the x-ray beam to the area of interest reduces scatter radiation and minimizes patient dose.
-
Filtration: Removing low-energy x-rays from the beam reduces skin dose without significantly affecting image quality.
-
Shielding: Using protective barriers (e.g., lead aprons, gonadal shields) to protect sensitive organs from radiation.
-
Technical Factors: Optimizing kVp and mAs settings to achieve adequate image quality with the lowest possible dose.
Conclusion: A Synergistic Blend of Physics and Imaging
Radiographic imaging is a powerful diagnostic tool that relies on a sophisticated interplay between physics and imaging science. Understanding the underlying principles of x-ray production, interaction with matter, image formation, and radiation protection is essential for radiographers to produce high-quality images while ensuring patient safety. As technology continues to evolve, a strong foundation in radiographic physics remains crucial for providing optimal patient care. The future of medical imaging will undoubtedly involve even more sophisticated techniques and technologies, but the core principles discussed here will continue to be fundamental to the field. Continual learning and adaptation are necessary for professionals in this constantly evolving field.
Latest Posts
Latest Posts
-
70 Miles Per Hour In Kilometers
May 20, 2025
-
How Many Hours Is 4 Days
May 20, 2025
-
How Many Kg Is 9 Stone
May 20, 2025
-
61 Kg In Stones And Lbs
May 20, 2025
-
58 0 Kg In Stones And Pounds
May 20, 2025
Related Post
Thank you for visiting our website which covers about Essentials Of Radiographic Physics And Imaging . We hope the information provided has been useful to you. Feel free to contact us if you have any questions or need further assistance. See you next time and don't miss to bookmark.