Draw The Major Organic Product Generated In The Reaction Below
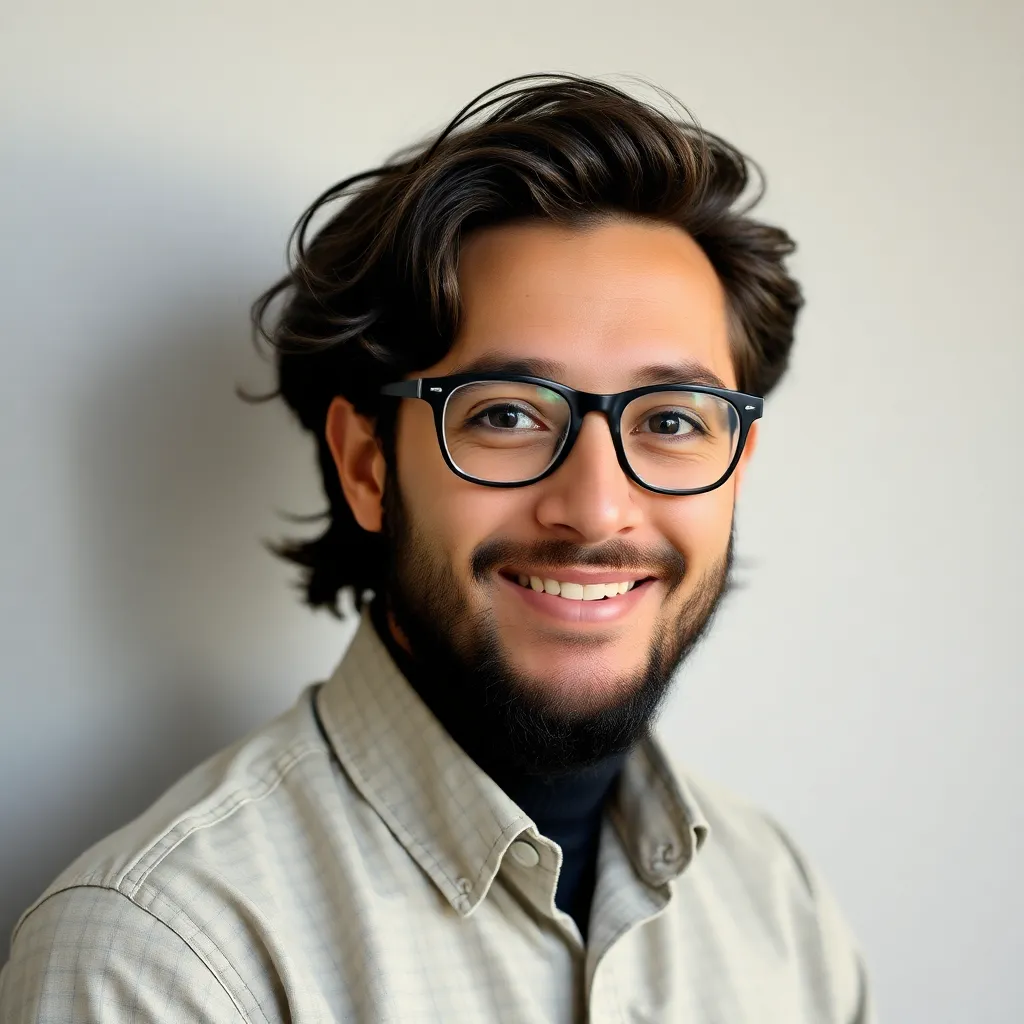
Holbox
May 08, 2025 · 6 min read
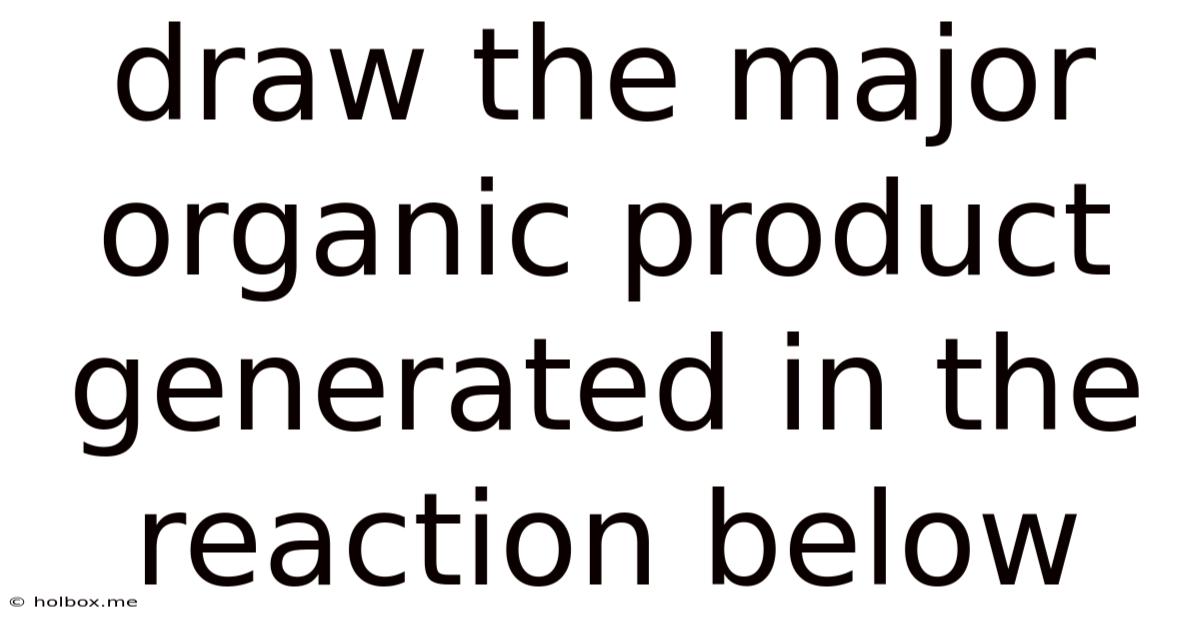
Table of Contents
- Draw The Major Organic Product Generated In The Reaction Below
- Table of Contents
- Drawing the Major Organic Product: A Deep Dive into Reaction Mechanisms and Prediction
- Understanding Reaction Mechanisms: The Foundation of Product Prediction
- Common Reaction Types and Product Prediction Strategies
- 1. Electrophilic Addition to Alkenes
- 2. Nucleophilic Substitution Reactions (SN1 and SN2)
- 3. Elimination Reactions (E1 and E2)
- 4. Electrophilic Aromatic Substitution
- Advanced Considerations in Product Prediction
- Practical Application: Step-by-Step Product Prediction
- Conclusion
- Latest Posts
- Related Post
Drawing the Major Organic Product: A Deep Dive into Reaction Mechanisms and Prediction
Predicting the major organic product of a reaction is a cornerstone of organic chemistry. It requires a thorough understanding of reaction mechanisms, functional group reactivity, and the influence of steric and electronic factors. This article delves into the process of accurately predicting the major product, providing a detailed explanation through several examples and highlighting crucial considerations. We'll explore various reaction types, focusing on how to identify the key steps and anticipate the most likely outcome.
Understanding Reaction Mechanisms: The Foundation of Product Prediction
Before we can confidently predict the major organic product, we must thoroughly grasp the underlying reaction mechanism. A reaction mechanism is a step-by-step description of how bonds break and form during a chemical transformation. Knowing the mechanism allows us to:
- Identify reactive intermediates: These are short-lived species formed during the reaction, like carbocations, carbanions, and radicals. Their stability significantly impacts the product distribution.
- Predict regioselectivity: This refers to the preference for reaction at one specific site over another on a molecule. For example, in electrophilic addition to alkenes, Markovnikov's rule dictates the regioselectivity.
- Predict stereoselectivity: This deals with the preference for forming one stereoisomer (e.g., enantiomer or diastereomer) over others. Understanding stereochemistry is critical for accurate product prediction, particularly in reactions involving chiral centers.
- Understand the rate-determining step: The slowest step in a multi-step mechanism governs the overall reaction rate and often influences the product outcome.
Common Reaction Types and Product Prediction Strategies
Several common reaction types will be examined, each with its own set of rules and considerations for predicting the major product.
1. Electrophilic Addition to Alkenes
Alkenes are electron-rich due to the presence of the pi bond. They undergo electrophilic addition reactions where an electrophile attacks the double bond, leading to the formation of a new sigma bond.
Mechanism: The reaction typically proceeds via a two-step mechanism involving a carbocation intermediate.
- Step 1: Electrophilic attack on the alkene, forming a carbocation.
- Step 2: Nucleophilic attack on the carbocation, leading to the final product.
Markovnikov's Rule: This rule states that the electrophile will add to the carbon atom with the fewer number of alkyl substituents (the less substituted carbon), resulting in the more stable carbocation intermediate.
Example: Addition of HBr to propene. The H+ adds to the less substituted carbon, forming a secondary carbocation, which is then attacked by Br-. The major product is 2-bromopropane.
2. Nucleophilic Substitution Reactions (SN1 and SN2)
These reactions involve the substitution of a leaving group by a nucleophile. Two main mechanisms exist: SN1 and SN2.
SN1 (Unimolecular Nucleophilic Substitution): This reaction proceeds through a carbocation intermediate. The rate is dependent only on the concentration of the substrate (alkyl halide).
- Step 1: Leaving group departs, forming a carbocation.
- Step 2: Nucleophile attacks the carbocation.
SN2 (Bimolecular Nucleophilic Substitution): This is a concerted mechanism; the nucleophile attacks the substrate simultaneously with the departure of the leaving group. The rate depends on the concentrations of both the substrate and the nucleophile. It proceeds with inversion of configuration at the stereocenter.
Factors influencing SN1 vs. SN2:
- Substrate: Tertiary substrates favor SN1, while primary substrates favor SN2. Secondary substrates can undergo both mechanisms.
- Nucleophile: Strong nucleophiles favor SN2, while weak nucleophiles favor SN1.
- Solvent: Polar protic solvents favor SN1, while polar aprotic solvents favor SN2.
Example: Reaction of 2-bromobutane with sodium hydroxide (NaOH). This reaction will proceed via an SN1 mechanism (although some SN2 may occur) due to the secondary substrate and the relatively weak nucleophile in the presence of a protic solvent (water from aqueous NaOH). The major product will be a mixture of 2-butanol and 1-butanol, with 2-butanol likely predominating due to the more stable carbocation intermediate.
3. Elimination Reactions (E1 and E2)
Elimination reactions involve the removal of a leaving group and a proton from adjacent carbon atoms, resulting in the formation of a double bond (alkene).
E1 (Unimolecular Elimination): Similar to SN1, this reaction proceeds through a carbocation intermediate. The rate depends only on the concentration of the substrate.
E2 (Bimolecular Elimination): This is a concerted mechanism where the base abstracts a proton and the leaving group departs simultaneously. The rate depends on the concentrations of both the substrate and the base.
Zaitsev's Rule: In elimination reactions, the major product is typically the more substituted alkene (the alkene with the most alkyl groups attached to the double bond), due to its greater stability.
Example: Dehydration of 2-methylcyclohexanol. This reaction, typically carried out with a strong acid catalyst like sulfuric acid, will proceed via an E1 mechanism. The major product will be 1-methylcyclohexene, in accordance with Zaitsev's rule, as it is the more substituted alkene.
4. Electrophilic Aromatic Substitution
Aromatic compounds undergo electrophilic aromatic substitution, where an electrophile replaces a hydrogen atom on the aromatic ring.
Mechanism: The reaction involves a two-step mechanism involving the formation of a resonance-stabilized carbocation intermediate (arenium ion).
Activating and Deactivating Groups: Substituents on the aromatic ring can influence the regioselectivity of the reaction. Activating groups (e.g., -OH, -NH2) direct the electrophile to the ortho and para positions, while deactivating groups (e.g., -NO2, -COOH) direct the electrophile to the meta position.
Example: Nitration of toluene. The methyl group is an activating group and directs the nitronium ion (NO2+) to the ortho and para positions. The para product will be the major product due to steric hindrance at the ortho position.
Advanced Considerations in Product Prediction
Beyond the fundamental reaction mechanisms, several other factors influence product formation:
- Steric hindrance: Bulky groups can hinder the approach of reactants, affecting both the rate and regioselectivity of the reaction.
- Electronic effects: Electron-donating and electron-withdrawing groups can influence the reactivity of the molecule.
- Temperature: Higher temperatures often favor elimination reactions over substitution reactions.
- Solvent effects: Solvents can influence the stability of intermediates and the reaction rate.
- Equilibrium considerations: In reversible reactions, the position of equilibrium can determine the major product.
Practical Application: Step-by-Step Product Prediction
Let's illustrate the process of predicting the major organic product with a specific example:
Reaction: Reaction of 2-chloro-2-methylbutane with potassium tert-butoxide (t-BuOK) in tert-butyl alcohol.
Analysis:
- Identify the reactants: 2-chloro-2-methylbutane (a tertiary alkyl halide) and potassium tert-butoxide (a strong, bulky base).
- Consider the reaction conditions: A strong base in a non-polar solvent suggests an elimination reaction (E2) is likely to dominate.
- Apply Zaitsev's rule: The major product will be the most substituted alkene, 2-methyl-2-butene. The bulky base (t-BuOK) also favors the less hindered elimination pathway leading to this product.
Therefore, the major organic product is 2-methyl-2-butene.
Conclusion
Accurately predicting the major organic product requires a comprehensive understanding of reaction mechanisms, functional group reactivity, and various influencing factors. By systematically analyzing the reactants, reaction conditions, and applying relevant rules (Markovnikov's rule, Zaitsev's rule, etc.), one can confidently determine the most probable outcome of a chemical transformation. Remember to always consider steric effects and electronic effects for a more refined prediction. Practice and experience are essential to mastering this skill, and this detailed approach provides a solid framework for success in organic chemistry.
Latest Posts
Related Post
Thank you for visiting our website which covers about Draw The Major Organic Product Generated In The Reaction Below . We hope the information provided has been useful to you. Feel free to contact us if you have any questions or need further assistance. See you next time and don't miss to bookmark.