Draw An Electron-dot Structure For Glycine
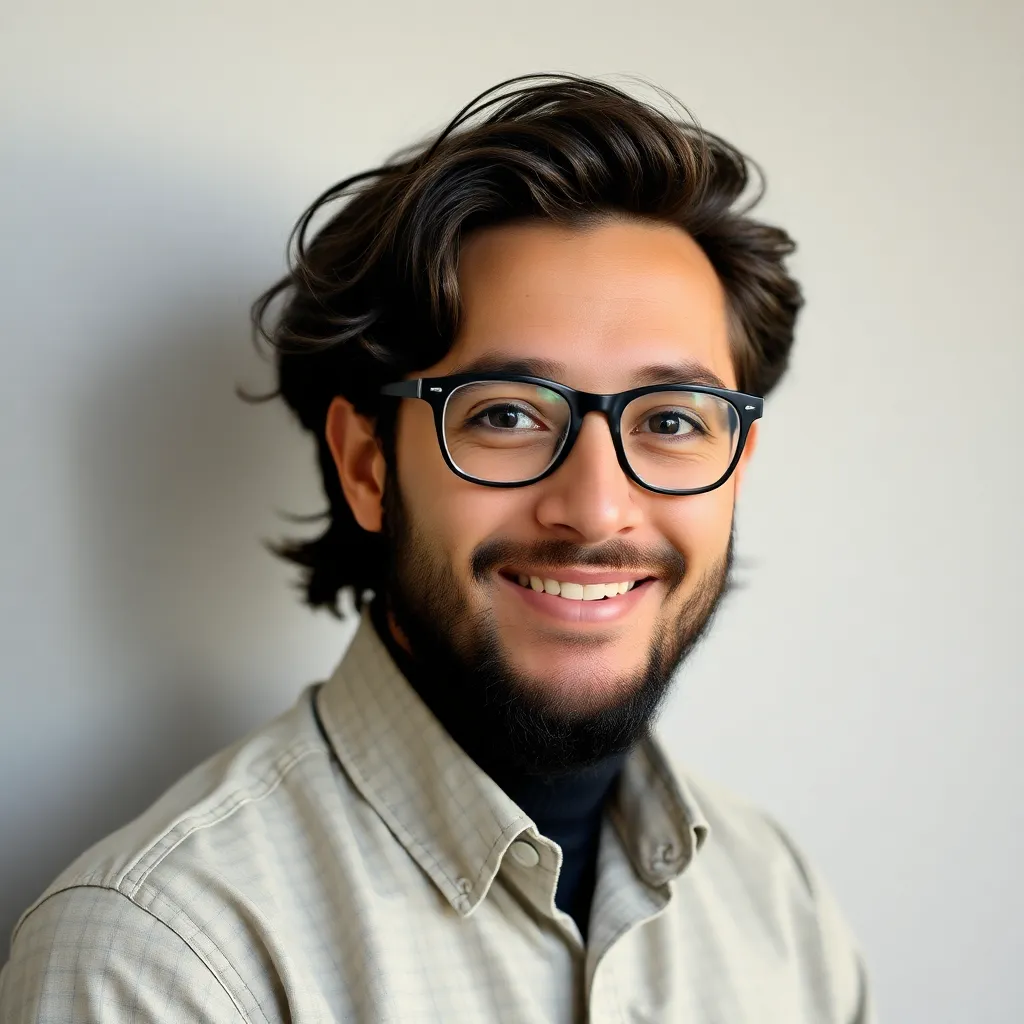
Holbox
May 10, 2025 · 6 min read
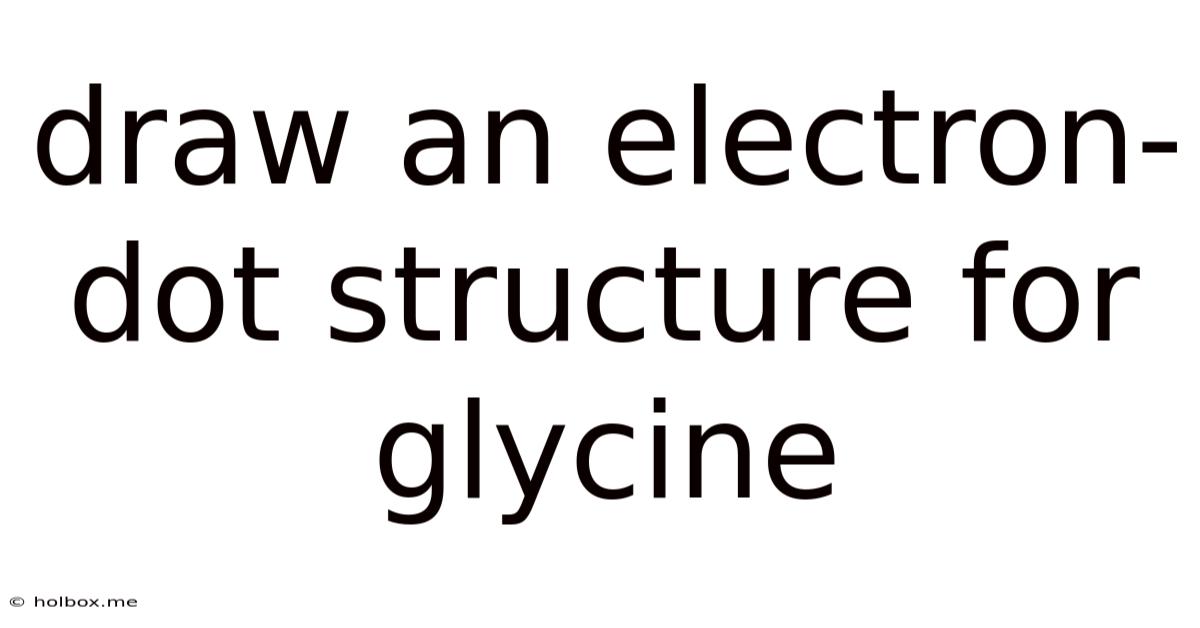
Table of Contents
- Draw An Electron-dot Structure For Glycine
- Table of Contents
- Drawing the Electron-Dot Structure for Glycine: A Comprehensive Guide
- Understanding the Basics: Valence Electrons and the Octet Rule
- Glycine's Chemical Formula: C₂H₅NO₂
- Step-by-Step Construction of Glycine's Electron-Dot Structure
- Understanding the Zwitterionic Form of Glycine
- Resonance Structures in Glycine
- Importance of Electron-Dot Structures in Biochemistry and Organic Chemistry
- Advanced Considerations: Formal Charges and Oxidation States
- Formal Charge Calculation
- Oxidation States
- Conclusion
- Latest Posts
- Related Post
Drawing the Electron-Dot Structure for Glycine: A Comprehensive Guide
Glycine, the simplest of the 20 proteinogenic amino acids, provides an excellent starting point for understanding the electron-dot structures of organic molecules. This comprehensive guide will walk you through the step-by-step process of drawing the electron-dot structure (also known as a Lewis structure) for glycine, explaining the underlying principles and considerations along the way. We will cover valence electrons, octet rule exceptions, and the importance of understanding these structures in the context of biochemistry and organic chemistry.
Understanding the Basics: Valence Electrons and the Octet Rule
Before diving into glycine, let's refresh our understanding of fundamental concepts. The electron-dot structure visually represents the valence electrons of atoms within a molecule. Valence electrons are the electrons in the outermost shell of an atom, which participate in chemical bonding. The octet rule states that atoms tend to gain, lose, or share electrons to achieve a stable configuration of eight valence electrons, similar to the electron configuration of a noble gas. This rule is crucial for predicting the bonding patterns in molecules.
Glycine's Chemical Formula: C₂H₅NO₂
Glycine's chemical formula, C₂H₅NO₂, tells us the types and numbers of atoms present in a single molecule. To draw the electron-dot structure, we need to determine the number of valence electrons contributed by each atom:
- Carbon (C): Group 14, 4 valence electrons
- Hydrogen (H): Group 1, 1 valence electron
- Nitrogen (N): Group 15, 5 valence electrons
- Oxygen (O): Group 16, 6 valence electrons
Therefore, the total number of valence electrons in glycine is: (2 × 4) + (5 × 1) + 5 + (2 × 6) = 26 valence electrons.
Step-by-Step Construction of Glycine's Electron-Dot Structure
Now, let's build the electron-dot structure systematically:
Step 1: Identify the central atom(s). In glycine, carbon atoms are usually the central atoms due to their ability to form multiple bonds.
Step 2: Connect the atoms with single bonds. Begin by connecting the atoms with single bonds (represented by lines), forming a skeletal structure. Remember to utilize the available atoms from the chemical formula. A basic skeletal structure is a good starting point:
H
|
H-N-C-C-O-H
| ||
H O
Step 3: Distribute the remaining valence electrons. We've used 14 electrons (7 single bonds × 2 electrons/bond) from the total 26. The remaining 12 electrons need to be distributed to satisfy the octet rule (or duet rule for hydrogen) for each atom. Start by placing lone pairs of electrons around the outer atoms (oxygen, nitrogen, and hydrogens).
Step 4: Check the octet rule. At this stage, some atoms might not have a complete octet. To achieve this, we often need to form double or triple bonds. In glycine, the carbonyl group (C=O) needs a double bond between carbon and oxygen to satisfy the octet rule for both atoms.
Step 5: Final Electron-Dot Structure: Following the steps outlined above, we arrive at the following electron-dot structure for glycine:
H
|
H-N-C-C=O
| ||
H O⁻
The negative charge on one of the oxygens and the overall neutral charge on the molecule. This zwitterionic form is a key feature of glycine in aqueous solutions and contributes to its amphoteric nature.
Understanding the Zwitterionic Form of Glycine
The electron-dot structure above illustrates the zwitterionic form of glycine. A zwitterion is a molecule that has both a positive and negative charge, but has an overall neutral charge. In glycine, the carboxyl group (-COOH) loses a proton (H⁺), becoming negatively charged (-COO⁻), while the amino group (-NH₂) gains a proton, becoming positively charged (-NH₃⁺). This zwitterionic form is prevalent in aqueous solutions at physiological pH.
Resonance Structures in Glycine
While the structure presented above accurately depicts the distribution of electrons, it's important to note that resonance exists within the carboxylate group (-COO⁻). Resonance describes the delocalization of electrons over multiple atoms. In the case of glycine, resonance structures can be drawn, indicating that the negative charge is shared equally between the two oxygen atoms:
H
|
H-N-C-C=O ↔ H-N-C-C-O⁻
| || | ||
H O⁻ H O
These resonance structures contribute to the stability of the carboxylate group.
Importance of Electron-Dot Structures in Biochemistry and Organic Chemistry
Understanding electron-dot structures is crucial for several reasons:
-
Predicting Molecular Geometry: The arrangement of electrons influences the geometry of a molecule, impacting its reactivity and properties. For instance, the tetrahedral geometry around the carbon atoms in glycine affects its interactions with other molecules.
-
Understanding Chemical Reactivity: Electron-dot structures help predict the reactivity of molecules. Lone pairs of electrons and the presence of double bonds indicate regions of high electron density, making them susceptible to electrophilic attack.
-
Analyzing Acid-Base Properties: The presence of the carboxyl and amino groups in glycine indicates its amphoteric nature (able to act as both an acid and a base), a critical property in biochemical processes. The electron-dot structure helps to visualize the protonation and deprotonation of these groups.
-
Drug Design and Development: Accurate depiction of electron distribution and charge is essential in the pharmaceutical industry for designing and optimizing drug molecules to interact effectively with biological targets.
Advanced Considerations: Formal Charges and Oxidation States
While the octet rule is a useful guideline, exceptions exist. Furthermore, calculating formal charges and oxidation states provides a more nuanced understanding of electron distribution within a molecule.
Formal Charge Calculation
Formal charge helps determine which atom in the molecule carries a partial positive or negative charge, often refining the electron-dot structure further.
Oxidation States
Oxidation states describe the hypothetical charge an atom would have if all bonds were completely ionic. Calculating oxidation states assists in understanding electron transfer processes and redox reactions, which are essential in many biological systems.
Conclusion
Drawing the electron-dot structure for glycine provides a practical application of fundamental concepts in chemistry. Understanding valence electrons, the octet rule, resonance structures, zwitterions, formal charges, and oxidation states allow for a deeper comprehension of glycine's structure, its properties, and its function in biological systems. This detailed analysis is not only applicable to glycine but can be extended to understanding the structural characteristics of other amino acids and organic molecules, highlighting the importance of these concepts in various scientific disciplines. The ability to accurately depict and interpret electron-dot structures empowers scientists to predict and manipulate the properties of molecules for diverse applications, ranging from drug development to materials science. This skill, therefore, is fundamental for any aspiring chemist or biochemist.
Latest Posts
Related Post
Thank you for visiting our website which covers about Draw An Electron-dot Structure For Glycine . We hope the information provided has been useful to you. Feel free to contact us if you have any questions or need further assistance. See you next time and don't miss to bookmark.