Draw A Resonance Structure That Places A Pi Bond
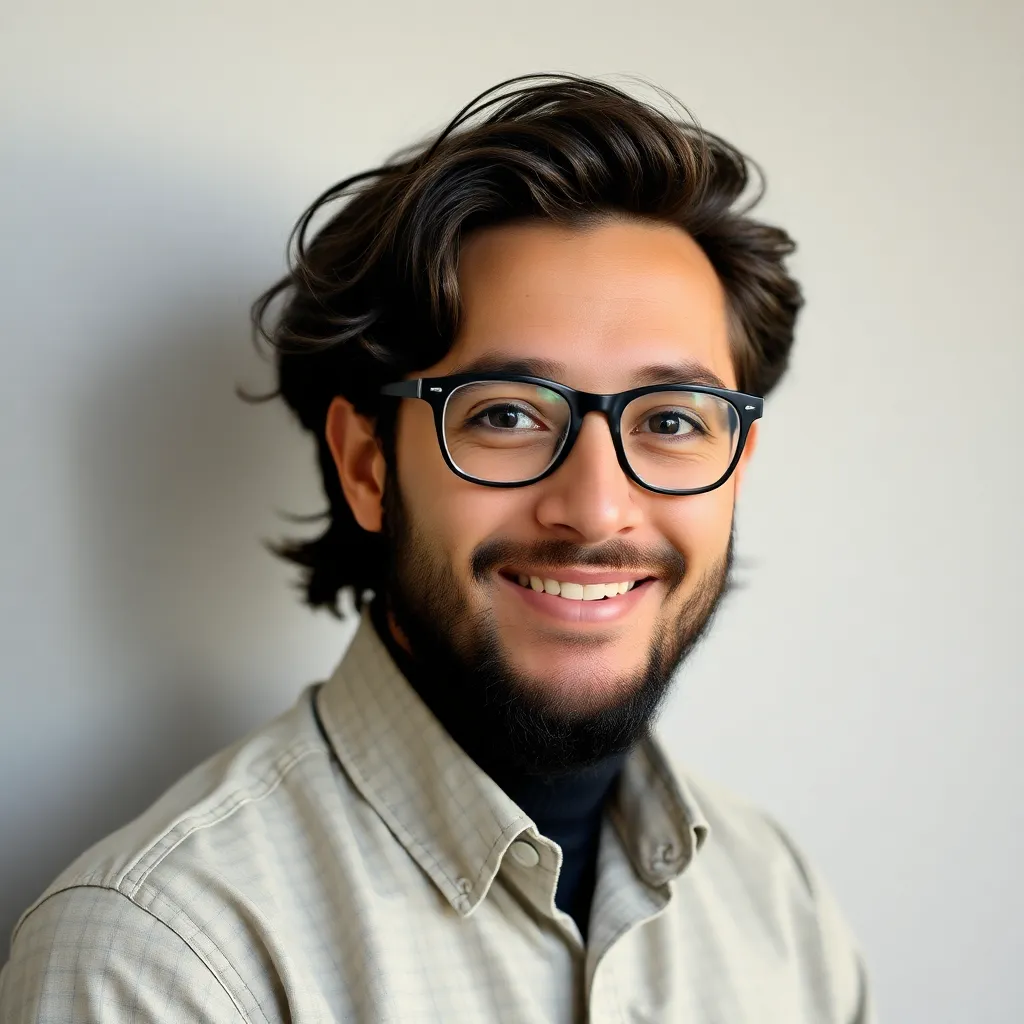
Holbox
Apr 01, 2025 · 6 min read
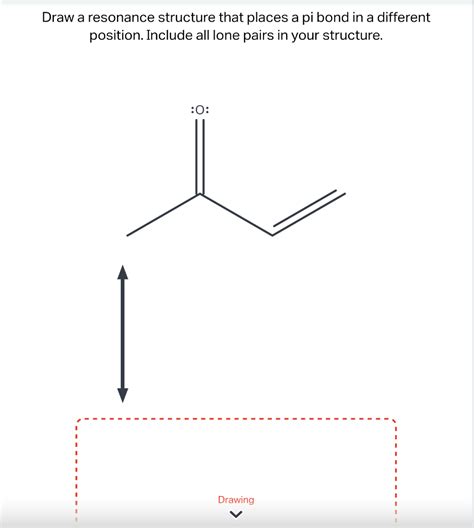
Table of Contents
- Draw A Resonance Structure That Places A Pi Bond
- Table of Contents
- Drawing Resonance Structures with Pi Bonds: A Comprehensive Guide
- Understanding Resonance and Delocalized Electrons
- Key Characteristics of Resonance Structures
- Steps to Draw Resonance Structures
- Examples of Drawing Resonance Structures with Pi Bonds
- Example 1: Nitrate Ion (NO₃⁻)
- Example 2: Benzene (C₆H₆)
- Example 3: Acetate Ion (CH₃COO⁻)
- Example 4: Allylic Cation
- Advanced Resonance Concepts
- Resonance Hybrid
- Major and Minor Contributors
- Resonance Energy
- Applications of Resonance
- Conclusion
- Latest Posts
- Latest Posts
- Related Post
Drawing Resonance Structures with Pi Bonds: A Comprehensive Guide
Resonance structures are crucial for understanding the delocalized electrons in molecules, particularly those containing pi bonds. Mastering their drawing is fundamental to organic chemistry and beyond. This comprehensive guide will walk you through the process, explaining the theory behind resonance and providing numerous examples to solidify your understanding.
Understanding Resonance and Delocalized Electrons
Before diving into drawing resonance structures, let's refresh our understanding of key concepts. Resonance describes a phenomenon where a single Lewis structure is insufficient to represent the true electron distribution within a molecule. Instead, the actual molecule is a hybrid of multiple contributing structures, called resonance structures or canonical forms.
The electrons responsible for resonance are typically delocalized electrons, meaning they aren't confined to a single atom or bond, but are spread across multiple atoms. These delocalized electrons are often found in pi bonds (π bonds) and lone pairs that are adjacent to pi bonds. A pi bond is formed by the sideways overlap of p-orbitals, creating an electron cloud above and below the sigma bond.
Key Characteristics of Resonance Structures
- Same Atom Connectivity: All resonance structures must have the same arrangement of atoms. Only the placement of electrons differs.
- Formal Charges: Formal charges can change between resonance structures, but the overall charge of the molecule remains the same.
- Equivalent Structures Contribute Equally: If resonance structures are equivalent (identical except for electron placement), they contribute equally to the overall resonance hybrid.
- Non-equivalent Structures Contribute Unequally: Non-equivalent resonance structures contribute differently. Structures with fewer formal charges and those with negative charges on more electronegative atoms are more stable and contribute more significantly.
Steps to Draw Resonance Structures
Let's outline a systematic approach to drawing resonance structures, particularly those involving pi bonds:
-
Identify the Pi Bonds and Lone Pairs: Begin by identifying all pi bonds (double or triple bonds) and lone pairs of electrons adjacent to these pi bonds. These are the electrons most likely involved in resonance.
-
Move Pi Electrons: Choose a pi bond (or a lone pair adjacent to a pi bond). Move one pair of pi electrons towards an adjacent atom. This will typically result in forming a new pi bond or completing an octet on an atom that previously lacked one.
-
Create New Pi Bonds or Lone Pairs: The movement of the electrons will create a new pi bond or shift a lone pair’s location.
-
Maintain Formal Charges: Carefully calculate the formal charge on each atom in the new resonance structure. Remember, the sum of formal charges must equal the overall charge of the molecule.
-
Repeat Steps 2-4: If possible, continue moving electrons to generate additional resonance structures. Try different electron movements to explore all possible contributing structures.
-
Evaluate Stability: Evaluate the relative stability of your resonance structures. The more stable structures contribute more significantly to the resonance hybrid. Factors contributing to stability include:
- Minimizing formal charges: Structures with fewer formal charges are more stable.
- Placing negative charges on more electronegative atoms: Negative charges are more stable on electronegative atoms (like oxygen or nitrogen).
- Avoiding separation of unlike charges: Structures with adjacent charges of the opposite sign are less stable.
- Complete octets (where applicable): Structures where more atoms have complete octets are generally more stable.
Examples of Drawing Resonance Structures with Pi Bonds
Let's illustrate the process with a few examples, focusing on the movement of pi electrons:
Example 1: Nitrate Ion (NO₃⁻)
The nitrate ion (NO₃⁻) is a classic example exhibiting resonance.
-
Initial Structure: Begin with one Lewis structure where one nitrogen-oxygen bond is a double bond.
-
Move Pi Electrons: Move the electrons from the nitrogen-oxygen double bond to form a lone pair on the oxygen. Simultaneously, move a lone pair from one of the other oxygens to form a new nitrogen-oxygen double bond.
-
Repeat: Repeat this process for all three oxygen atoms.
You'll end up with three equivalent resonance structures. The actual nitrate ion is a resonance hybrid where the negative charge is delocalized across all three oxygen atoms.
Example 2: Benzene (C₆H₆)
Benzene is another prominent example where pi bond resonance is crucial.
-
Initial Structure: Start with one Kekule structure of benzene, which shows alternating single and double bonds.
-
Move Pi Electrons: Move the pi electrons from one double bond to form a new double bond in the adjacent position.
-
Repeat: Continue this process, generating two equivalent resonance structures which are usually depicted as a circle within the hexagon to represent the delocalized electron cloud.
Example 3: Acetate Ion (CH₃COO⁻)
The acetate ion showcases resonance involving a pi bond and a lone pair.
-
Initial Structure: Start with the Lewis structure showing one carbon-oxygen double bond and one carbon-oxygen single bond. The negative charge is located on the singly-bonded oxygen.
-
Move Pi Electrons & Lone Pairs: Move the pi electrons from the carbon-oxygen double bond to create a lone pair on the oxygen. Simultaneously, move a lone pair from the singly-bonded oxygen to form a new pi bond with the carbon atom.
The resulting resonance structures show a delocalization of the negative charge between the two oxygen atoms.
Example 4: Allylic Cation
Allylic carbocations, like the allyl cation (CH₂=CH-CH₂⁺), exhibit resonance stabilization.
-
Initial Structure: The positive charge is initially localized on one carbon atom.
-
Move Pi Electrons: The pi electrons from the double bond can shift to form a new double bond involving the carbon with the positive charge, resulting in a new positive charge on the terminal carbon. This process can be reversed to generate two equivalent resonance structures.
This delocalization of the positive charge across two carbon atoms significantly stabilizes the allyl cation compared to a typical carbocation.
Advanced Resonance Concepts
Resonance Hybrid
The actual molecule isn't represented by any single resonance structure but rather by a resonance hybrid. This hybrid is a weighted average of all contributing resonance structures, with more stable structures contributing more heavily.
Major and Minor Contributors
Non-equivalent resonance structures contribute unequally. The most stable structures are the major contributors, while less stable structures are minor contributors.
Resonance Energy
Resonance stabilization results in a lower energy state for the molecule compared to any single resonance structure. This difference in energy is called resonance energy. Molecules with extensive resonance stabilization are generally more stable.
Applications of Resonance
Understanding resonance has wide-ranging applications in chemistry:
- Predicting Reactivity: Resonance can predict the reactivity of molecules. For instance, the delocalized electrons in benzene make it less reactive towards electrophilic addition reactions compared to alkenes.
- Explaining Molecular Properties: Resonance explains various properties like bond lengths, bond energies and molecular stability.
- Spectroscopy: Resonance has implications for interpreting spectroscopic data, such as NMR and UV-Vis spectroscopy.
- Drug Design: Understanding resonance is important in drug design as it influences the properties and interactions of drug molecules.
Conclusion
Drawing resonance structures is a fundamental skill in chemistry. By systematically moving electrons and evaluating the stability of resulting structures, you can effectively represent the delocalized electrons in molecules and gain insights into their properties and reactivity. This guide provides a comprehensive approach, combining theoretical explanations with practical examples to enhance your understanding and mastery of this essential chemical concept. Practicing drawing various resonance structures will build confidence and accuracy in this critical aspect of organic chemistry. Remember to pay close attention to formal charges and strive to identify the most stable contributing structures to accurately represent the resonance hybrid.
Latest Posts
Latest Posts
-
According To The Efficient Market Hypothesis
Apr 05, 2025
-
Comparisons Of Financial Data Made Within A Company Are Called
Apr 05, 2025
-
The Components Of Global Market Assessment Include
Apr 05, 2025
-
Foundations For Teaching English Language Learners
Apr 05, 2025
-
Fraudulent Reporting By Management Could Include
Apr 05, 2025
Related Post
Thank you for visiting our website which covers about Draw A Resonance Structure That Places A Pi Bond . We hope the information provided has been useful to you. Feel free to contact us if you have any questions or need further assistance. See you next time and don't miss to bookmark.