Consider The Following Reaction At 298 K
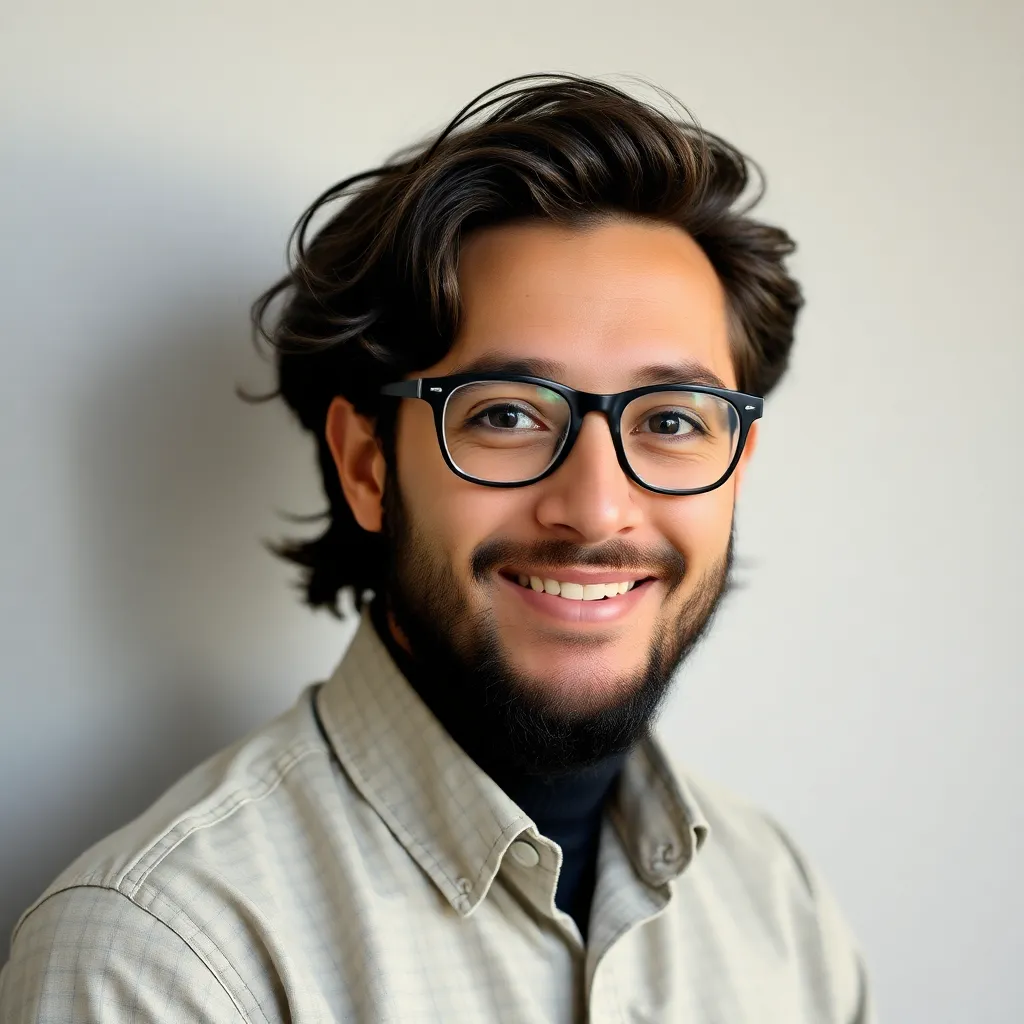
Holbox
Apr 27, 2025 · 6 min read
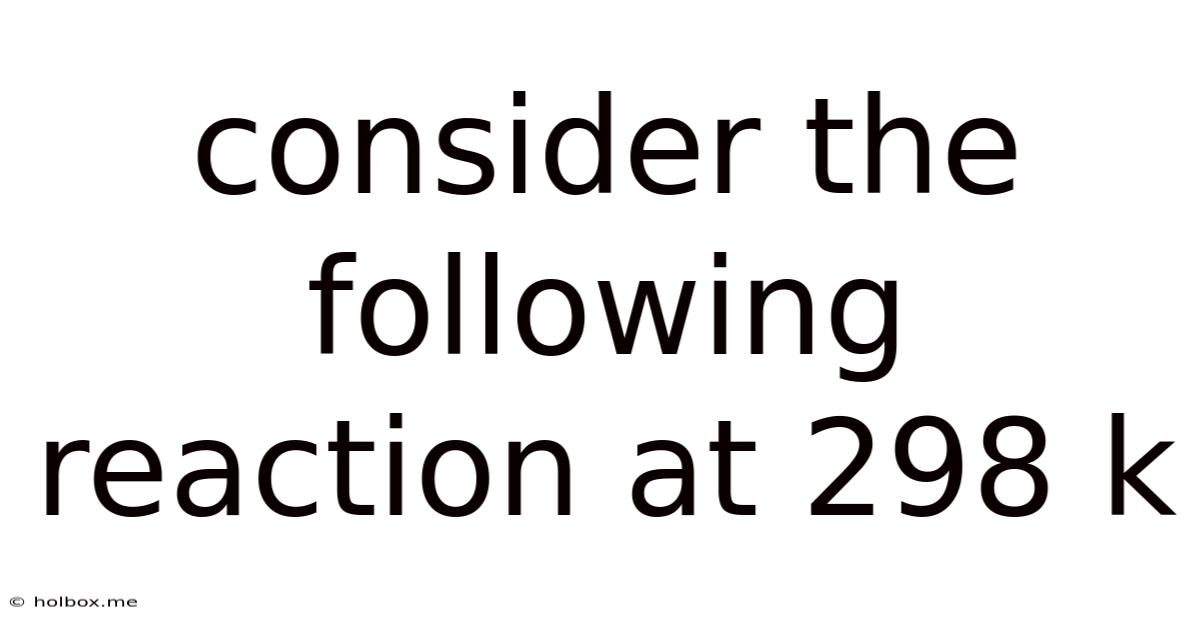
Table of Contents
- Consider The Following Reaction At 298 K
- Table of Contents
- Considering the Following Reaction at 298K: A Deep Dive into Thermodynamics and Kinetics
- Understanding Thermodynamics: The Driving Force Behind Reactions
- Understanding Kinetics: The Speed of Reactions
- Equilibrium at 298K: A Dynamic Balance
- Practical Applications and Considerations at 298K
- Conclusion
- Latest Posts
- Latest Posts
- Related Post
Considering the Following Reaction at 298K: A Deep Dive into Thermodynamics and Kinetics
This article explores the intricacies of chemical reactions at a constant temperature of 298K (25°C), a common standard condition in chemistry. We'll delve into the fundamental principles of thermodynamics and kinetics, focusing on how these principles govern the reaction's spontaneity, rate, and equilibrium. While a specific reaction isn't provided in the prompt, the principles discussed apply universally. Think of this as a foundational guide applicable to any reaction you might encounter at 298K.
Understanding Thermodynamics: The Driving Force Behind Reactions
Thermodynamics dictates whether a reaction will occur spontaneously. It focuses on the energy changes associated with the reaction, primarily through enthalpy (ΔH) and entropy (ΔS).
1. Enthalpy (ΔH): The Heat of Reaction
Enthalpy change (ΔH) represents the heat absorbed or released during a reaction at constant pressure.
-
Exothermic Reactions (ΔH < 0): These reactions release heat to the surroundings, resulting in a decrease in the system's enthalpy. They are often favored thermodynamically because they lower the overall energy of the system. Examples include combustion reactions and many neutralization reactions.
-
Endothermic Reactions (ΔH > 0): These reactions absorb heat from the surroundings, increasing the system's enthalpy. They require an input of energy to proceed. Examples include the melting of ice and many decomposition reactions.
2. Entropy (ΔS): The Measure of Disorder
Entropy (ΔS) measures the randomness or disorder of a system. Reactions tend to proceed spontaneously towards a state of higher entropy.
-
Increase in Entropy (ΔS > 0): This occurs when the products are more disordered than the reactants. For example, a reaction that produces more gas molecules than it consumes will generally have a positive entropy change.
-
Decrease in Entropy (ΔS < 0): This happens when the products are more ordered than the reactants. Such reactions are less favorable entropically. An example would be the polymerization of small molecules into a large polymer chain.
3. Gibbs Free Energy (ΔG): The Decisive Factor
The Gibbs Free Energy change (ΔG) combines enthalpy and entropy to predict the spontaneity of a reaction at a constant temperature and pressure:
ΔG = ΔH - TΔS
where:
-
ΔG is the Gibbs Free Energy change
-
ΔH is the enthalpy change
-
T is the temperature in Kelvin (298K in our case)
-
ΔS is the entropy change
-
ΔG < 0 (Negative): The reaction is spontaneous under the given conditions.
-
ΔG > 0 (Positive): The reaction is non-spontaneous under the given conditions. It would require an input of energy to proceed.
-
ΔG = 0 (Zero): The reaction is at equilibrium; the forward and reverse reaction rates are equal.
At 298K, the temperature term (T) significantly influences the spontaneity of reactions with small enthalpy changes. A reaction with a small positive ΔH but a large positive ΔS might still be spontaneous at 298K because the TΔS term outweighs the ΔH term.
Understanding Kinetics: The Speed of Reactions
While thermodynamics predicts if a reaction will occur, kinetics determines how fast it will occur. Several factors influence the reaction rate:
1. Activation Energy (Ea): The Energy Barrier
All reactions require an initial input of energy to overcome the activation energy barrier (Ea). This is the minimum energy required for reactants to collide effectively and form products. A higher activation energy results in a slower reaction rate.
2. Collision Theory: The Importance of Collisions
Reactions occur through collisions between reactant molecules. The rate of reaction depends on the frequency and effectiveness of these collisions. Several factors influence collision frequency and effectiveness:
- Concentration: Higher concentrations lead to more frequent collisions.
- Temperature: Higher temperatures increase the kinetic energy of molecules, leading to more frequent and energetic collisions.
- Surface Area: For heterogeneous reactions (involving reactants in different phases), a larger surface area increases the number of collisions.
- Catalyst: Catalysts provide an alternative reaction pathway with a lower activation energy, thus increasing the reaction rate without being consumed in the reaction.
3. Rate Laws and Rate Constants
The rate law expresses the relationship between the reaction rate and the concentrations of reactants. For a simple reaction A + B → C, the rate law might be:
Rate = k[A]<sup>m</sup>[B]<sup>n</sup>
where:
- k is the rate constant (temperature-dependent)
- [A] and [B] are the concentrations of reactants A and B
- m and n are the reaction orders with respect to A and B, respectively (determined experimentally)
The rate constant (k) is crucial for determining the reaction's speed. It increases with temperature according to the Arrhenius equation:
k = Ae<sup>-Ea/RT</sup>
where:
- A is the pre-exponential factor (frequency factor)
- Ea is the activation energy
- R is the gas constant
- T is the temperature in Kelvin
At 298K, a higher rate constant indicates a faster reaction. The Arrhenius equation highlights the strong temperature dependence of reaction rates. A small increase in temperature can significantly accelerate a reaction.
4. Reaction Mechanisms: The Step-by-Step Process
Many reactions proceed through a series of elementary steps called a reaction mechanism. Understanding the mechanism is crucial for manipulating the reaction rate. Rate-determining steps (the slowest step in the mechanism) dictate the overall reaction rate.
Equilibrium at 298K: A Dynamic Balance
Once a reaction reaches equilibrium at 298K, the forward and reverse reaction rates become equal. The concentrations of reactants and products remain constant, although the reaction continues at the microscopic level.
The equilibrium constant (K) describes the ratio of product concentrations to reactant concentrations at equilibrium:
K = [Products]<sup>p</sup> / [Reactants]<sup>r</sup>
where p and r are the stoichiometric coefficients of products and reactants, respectively. A large K value indicates that the equilibrium lies predominantly towards the products (favors product formation). A small K value indicates that the equilibrium favors the reactants.
The relationship between ΔG and K is given by:
ΔG° = -RTlnK
where ΔG° is the standard Gibbs Free Energy change at 298K and 1 atm pressure. This equation links thermodynamics (ΔG°) and equilibrium (K). A negative ΔG° corresponds to a K value greater than 1 (products favored), while a positive ΔG° corresponds to a K value less than 1 (reactants favored).
Practical Applications and Considerations at 298K
Many industrial processes and biological reactions operate near 298K. Understanding reaction thermodynamics and kinetics at this temperature is crucial for:
- Optimizing Reaction Conditions: Adjusting concentrations, temperature, and pressure to maximize product yield and reaction rate.
- Catalyst Development: Designing catalysts to lower activation energy and accelerate desired reactions.
- Predicting Reaction Outcomes: Using thermodynamic principles to predict whether a reaction will be spontaneous under specific conditions.
- Understanding Biological Systems: Analyzing enzymatic reactions and metabolic pathways that occur within living organisms at body temperature (approximately 298K).
Conclusion
Considering reactions at 298K requires a comprehensive understanding of both thermodynamics and kinetics. While thermodynamics determines the spontaneity of a reaction based on enthalpy and entropy changes, kinetics governs its speed through activation energy, collision theory, and reaction mechanisms. The equilibrium constant (K) quantifies the extent to which a reaction proceeds towards product formation at equilibrium. By integrating these principles, we can accurately predict and manipulate the behavior of countless chemical reactions at this standard temperature, paving the way for advancements in various fields, from industrial chemistry to biological sciences. Further study into specific reaction types will enhance your ability to apply these principles to real-world scenarios and develop a deeper understanding of chemical processes.
Latest Posts
Latest Posts
-
Consider The National Market For In Home Childcare
May 08, 2025
-
Dimethylallyl Pyrophosphate Serves As The Starting Point
May 08, 2025
-
Molar Mass Of Fe No3 3
May 08, 2025
-
Print Reading For Industry 11th Edition Pdf
May 08, 2025
-
Draw The Organic Products Formed In The Reaction Shown
May 08, 2025
Related Post
Thank you for visiting our website which covers about Consider The Following Reaction At 298 K . We hope the information provided has been useful to you. Feel free to contact us if you have any questions or need further assistance. See you next time and don't miss to bookmark.