Complete The Electron Pushing Mechanism For The Given Ether Synthesis
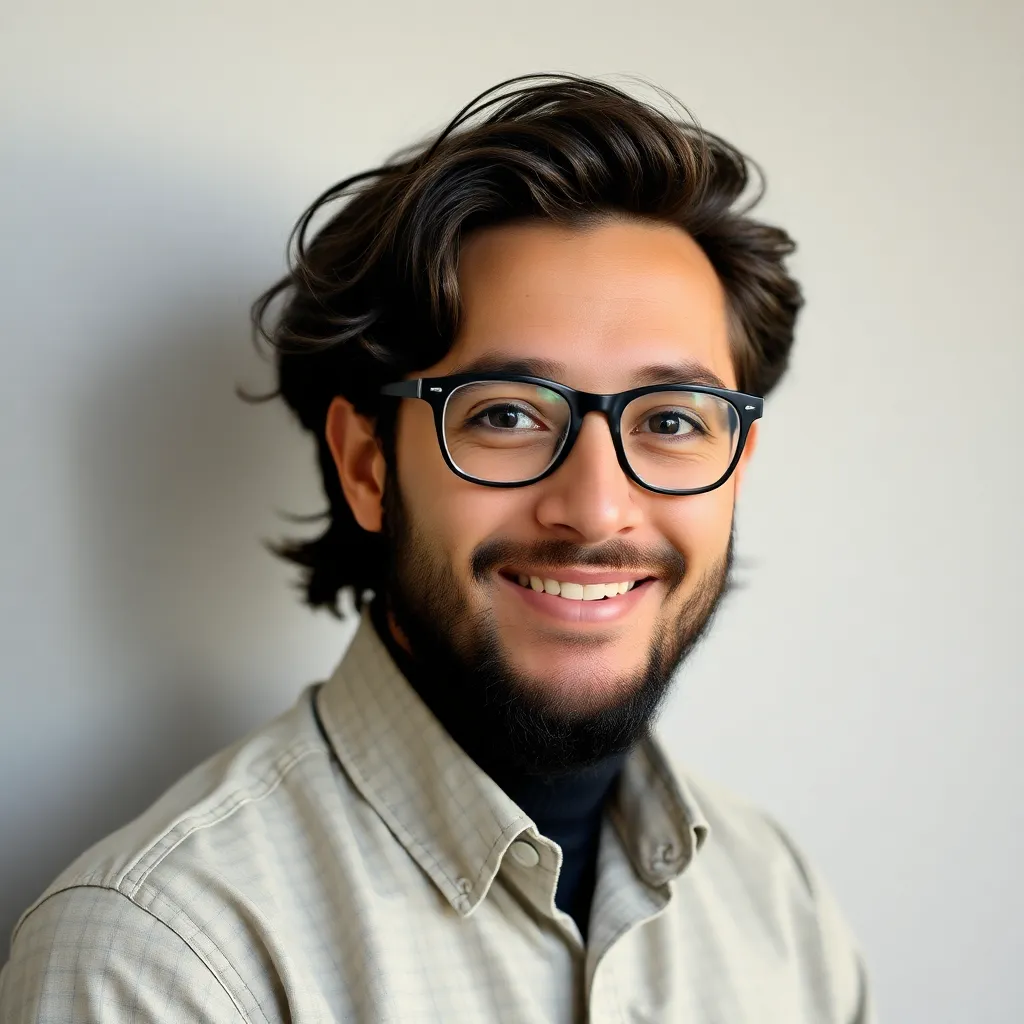
Holbox
Mar 29, 2025 · 6 min read
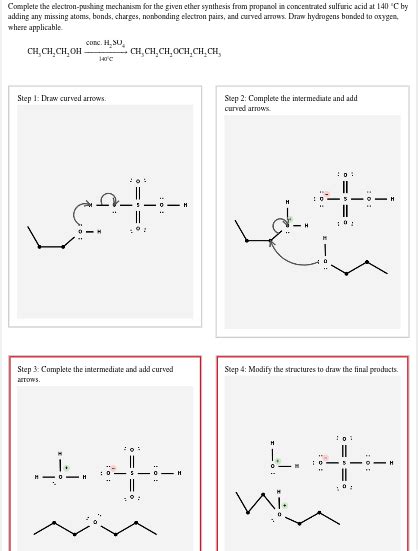
Table of Contents
- Complete The Electron Pushing Mechanism For The Given Ether Synthesis
- Table of Contents
- Decoding the Electron-Pushing Mechanism: A Deep Dive into Ether Synthesis
- Common Methods for Ether Synthesis and Their Mechanisms
- 1. Williamson Ether Synthesis: A Classic Approach
- 2. Acid-Catalyzed Dehydration of Alcohols: A Concerted Effort
- 3. Alkoxymercuration-Demercuration: A Mercury-Mediated Approach
- Understanding Electron Pushing: A Foundation for Mechanism Prediction
- Advanced Considerations and Practical Applications
- Conclusion: Mastering the Art of Ether Synthesis
- Latest Posts
- Latest Posts
- Related Post
Decoding the Electron-Pushing Mechanism: A Deep Dive into Ether Synthesis
Ether synthesis, a cornerstone reaction in organic chemistry, involves the formation of a carbon-oxygen-carbon (C-O-C) bond. While seemingly straightforward, understanding the underlying electron-pushing mechanisms is crucial for predicting reaction outcomes and designing efficient synthetic strategies. This article provides a comprehensive exploration of the electron-pushing mechanisms involved in various ether synthesis methods, focusing on clarity and detail for a thorough understanding. We'll move beyond simply stating the steps and delve into the nuances of each mechanism, explaining the driving forces behind each electron movement.
Common Methods for Ether Synthesis and Their Mechanisms
Several methods exist for synthesizing ethers, each with its unique mechanism. We'll examine the most prevalent:
1. Williamson Ether Synthesis: A Classic Approach
The Williamson ether synthesis is arguably the most widely used method for preparing symmetrical and unsymmetrical ethers. It involves the reaction of an alkoxide ion (RO⁻) with a primary alkyl halide (R'X) via an SN2 mechanism.
Mechanism:
-
Alkoxide Formation: The first step involves the formation of the alkoxide ion. This typically occurs through the deprotonation of an alcohol using a strong base such as sodium hydride (NaH) or potassium tert-butoxide (t-BuOK). The strong base abstracts the acidic proton from the alcohol, generating the nucleophilic alkoxide ion. The electron pushing here involves the lone pair on the base attacking the hydrogen of the alcohol, and the bond electrons moving onto the oxygen to form the negatively charged alkoxide.
R-O-H + NaH --> R-O⁻Na⁺ + H₂
-
SN2 Attack: The alkoxide ion then acts as a nucleophile, attacking the carbon atom bearing the leaving group (halide) in an SN2 reaction. The nucleophile's lone pair attacks the carbon from the backside, causing inversion of configuration at the stereocenter (if present). Simultaneously, the leaving group departs, taking its bonding electrons with it. This is the crucial step where the C-O bond is formed.
R-O⁻ + R'-X --> R-O-R' + X⁻
Factors Influencing Williamson Ether Synthesis:
- Steric hindrance: The SN2 mechanism is sensitive to steric hindrance. Bulky alkyl halides or alkoxides react more slowly or may not react at all. Primary alkyl halides are preferred for optimal yields.
- Leaving group ability: Good leaving groups (e.g., I⁻, Br⁻, Cl⁻) are essential for efficient SN2 reactions.
- Base choice: The choice of base influences the rate of alkoxide formation and can impact side reactions.
2. Acid-Catalyzed Dehydration of Alcohols: A Concerted Effort
This method is suitable for the synthesis of symmetrical ethers. Two molecules of alcohol react in the presence of an acid catalyst (e.g., sulfuric acid) to eliminate water and form the ether.
Mechanism:
-
Protonation: The acid catalyst protonates one of the alcohol molecules, making it a better leaving group. The electron pushing shows the oxygen of the alcohol picking up a proton from the acid, becoming positively charged.
R-O-H + H⁺ --> R-O⁺H₂
-
Nucleophilic Attack: A second alcohol molecule acts as a nucleophile, attacking the protonated alcohol. The electrons from the oxygen of the second alcohol attack the carbon, forming a new C-O bond.
R-O⁺H₂ + R-O-H --> R-O⁺H₂-O-R + H₂O
-
Proton Transfer and Deprotonation: A proton is transferred from the positively charged oxygen to form a water molecule. Finally, the conjugate base of the acid catalyst abstracts a proton from the oxonium ion to give the ether product and regenerate the catalyst.
R-O⁺H₂-O-R --> R-O-R + H₃O⁺
Limitations:
This method is less versatile than the Williamson ether synthesis, particularly for unsymmetrical ethers. It's susceptible to rearrangements, especially with secondary and tertiary alcohols.
3. Alkoxymercuration-Demercuration: A Mercury-Mediated Approach
This method uses mercury(II) acetate (Hg(OAc)₂), followed by reduction with a reducing agent such as sodium borohydride (NaBH₄), to add an alkoxy group to an alkene.
Mechanism:
-
Mercuration: The reaction begins with the electrophilic addition of mercury(II) acetate to the alkene. The π electrons of the alkene attack the mercury, forming a three-membered ring (mercurinium ion) intermediate. The acetate acts as the nucleophile, attacking the more substituted carbon of the mercurinium ion.
-
Alkoxide Attack: The alkoxide ion attacks the more substituted carbon of the mercurinium ion, resulting in the formation of a carbon-oxygen bond.
-
Demercuration: The mercury is then removed using a reducing agent like sodium borohydride (NaBH₄), replacing the mercury with a hydrogen. This step yields the ether product.
Advantages and Disadvantages:
The alkoxymercuration-demercuration method is regioselective, favoring Markovnikov addition. However, the use of mercury compounds raises environmental concerns, limiting its practical applications in modern synthesis.
Understanding Electron Pushing: A Foundation for Mechanism Prediction
The success of predicting reaction pathways hinges on mastering electron-pushing formalism. This involves depicting the movement of electrons using curved arrows, highlighting the flow of electron density within the molecules. The key is to understand that electrons always move in pairs.
- Lone pairs: Lone pairs on atoms are often involved in nucleophilic attacks, where they donate electron density to form new bonds.
- Pi bonds: Pi bonds (double or triple bonds) can act as nucleophiles or be broken to form new bonds.
- Bond formation: A curved arrow points from the electron source (nucleophile) to the electron acceptor (electrophile).
- Bond breaking: A curved arrow points from the bond being broken to the atom that receives the electron pair.
Advanced Considerations and Practical Applications
While the mechanisms discussed above provide a solid foundation, several factors can influence the efficiency and selectivity of ether synthesis. These include:
- Solvent effects: The choice of solvent significantly impacts reaction rates and selectivity. Polar aprotic solvents are frequently preferred for SN2 reactions.
- Temperature: Reaction temperature plays a crucial role in controlling reaction rates and minimizing side reactions.
- Catalyst optimization: Careful selection and optimization of catalysts can improve yields and selectivity.
Ether synthesis finds extensive applications in various fields, including:
- Pharmaceutical industry: Ethers are prevalent structural motifs in many pharmaceuticals and are commonly used as protecting groups.
- Materials science: Ethers are used as solvents and in the synthesis of polymers and other materials.
- Organic synthesis: Ethers are versatile building blocks, used in numerous organic transformations.
Conclusion: Mastering the Art of Ether Synthesis
Understanding the electron-pushing mechanisms underpinning ether synthesis is paramount for success in organic chemistry. This article has provided a detailed exploration of the most common methods, highlighting the crucial electron movements and factors that influence reaction outcomes. By mastering these mechanisms, you can confidently predict reaction pathways, design efficient synthetic routes, and tackle the challenges of more complex ether syntheses. The ability to visualize electron flow is a cornerstone skill, enabling you to not only understand existing reactions but to also design new ones. Continuous practice and a keen eye for detail are essential in mastering this crucial aspect of organic chemistry. Remember, the careful consideration of steric effects, leaving group ability, and the appropriate choice of reagents and conditions will ultimately determine the success of your ether synthesis endeavor.
Latest Posts
Latest Posts
-
Happy Go Lucky Electric Company Is The Only Company
Apr 02, 2025
-
Mrs Gonzalez Is Enrolled In Original Medicare
Apr 02, 2025
-
Match The Type Of Reflex With Its Description
Apr 02, 2025
-
Why Is The Term Experimental Psychologist Considered Somewhat Misleading
Apr 02, 2025
-
What Is The Meaning Of Darwins Expression Descent With Modification
Apr 02, 2025
Related Post
Thank you for visiting our website which covers about Complete The Electron Pushing Mechanism For The Given Ether Synthesis . We hope the information provided has been useful to you. Feel free to contact us if you have any questions or need further assistance. See you next time and don't miss to bookmark.