Choose The Correct Shape For Each Molecule
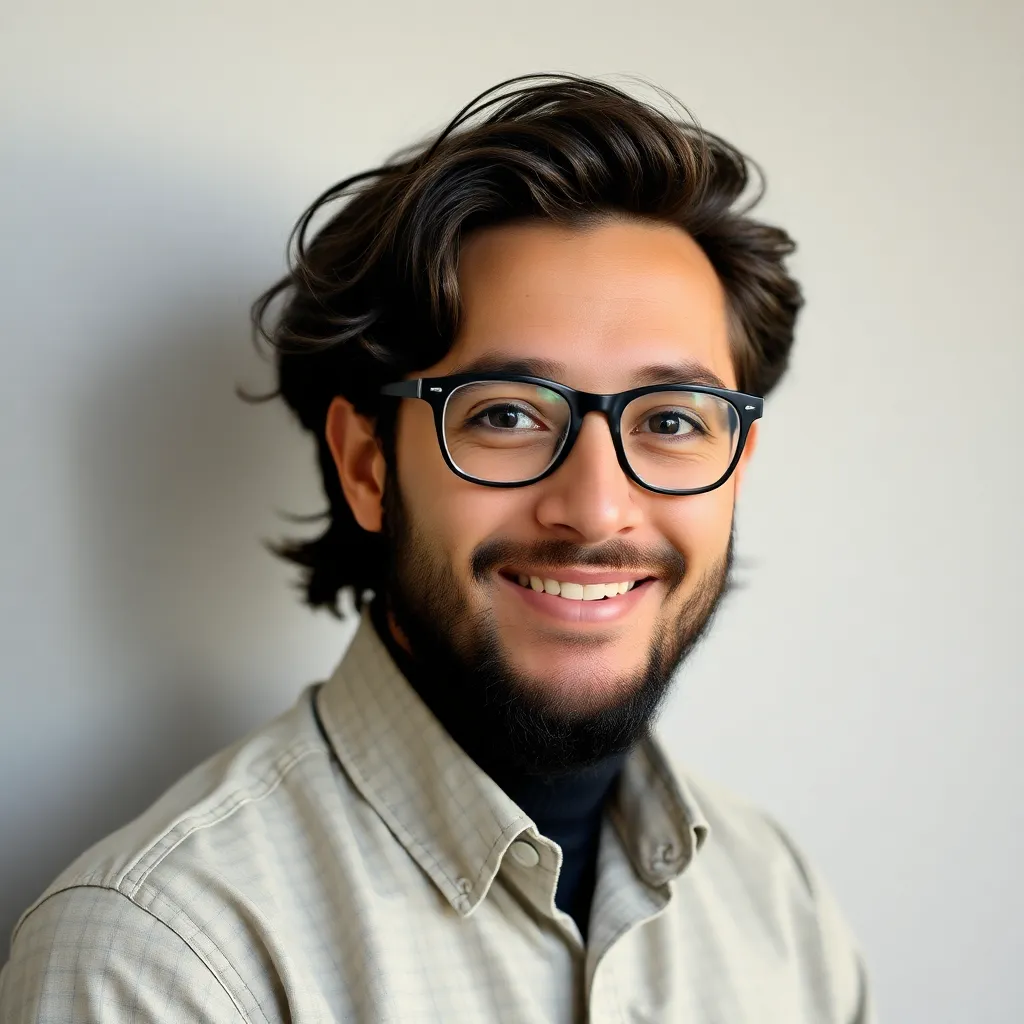
Holbox
May 11, 2025 · 6 min read
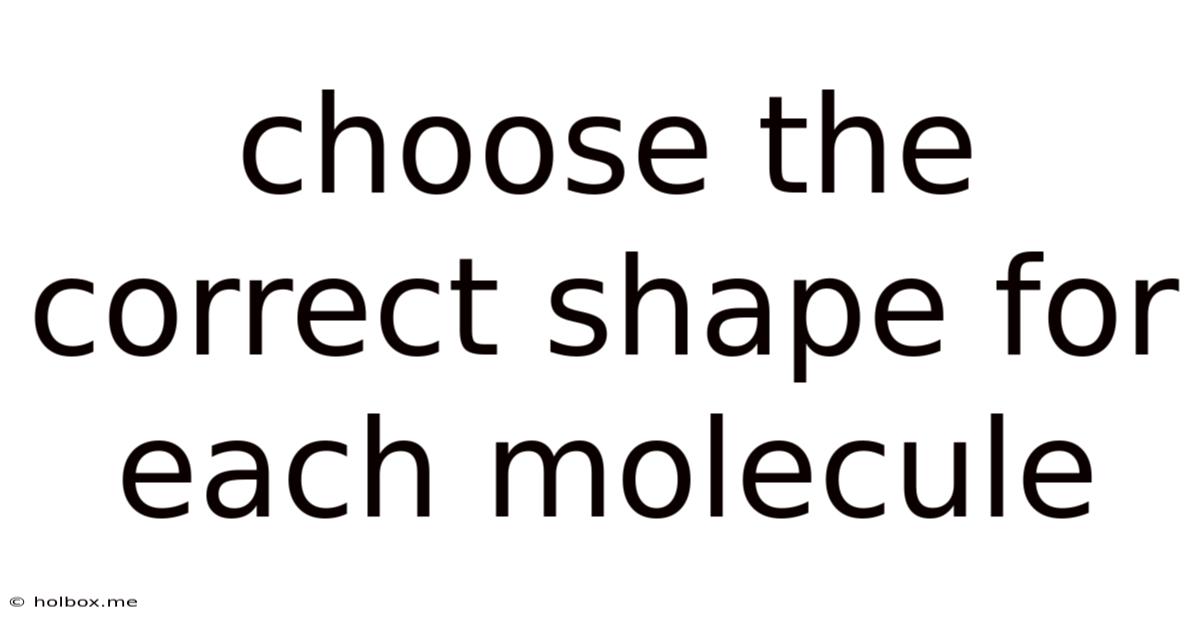
Table of Contents
- Choose The Correct Shape For Each Molecule
- Table of Contents
- Choosing the Correct Shape for Each Molecule: A Comprehensive Guide
- VSEPR Theory: The Foundation of Molecular Geometry
- Understanding Electron Domains
- Predicting Molecular Shapes Based on Electron Domains
- Applying VSEPR Theory: Step-by-Step Examples
- Example 1: Carbon Dioxide (CO₂)
- Example 2: Ammonia (NH₃)
- Example 3: Sulfur Hexafluoride (SF₆)
- Beyond VSEPR: Hybridization and Molecular Orbital Theory
- Hybridization
- Molecular Orbital Theory
- Factors Influencing Molecular Shape: Exceptions to VSEPR
- Advanced Techniques for Determining Molecular Shape
- The Importance of Molecular Shape in Chemistry and Beyond
- Conclusion
- Latest Posts
- Latest Posts
- Related Post
Choosing the Correct Shape for Each Molecule: A Comprehensive Guide
Determining the correct three-dimensional shape of a molecule is crucial in chemistry. Molecular shape dictates a molecule's properties, including its reactivity, polarity, and physical state. Understanding the principles of molecular geometry allows us to predict these properties and understand the behavior of substances at a fundamental level. This comprehensive guide will delve into the key concepts and techniques for choosing the correct shape for each molecule.
VSEPR Theory: The Foundation of Molecular Geometry
The Valence Shell Electron Pair Repulsion (VSEPR) theory is the cornerstone of predicting molecular shapes. This theory postulates that electron pairs, both bonding and non-bonding (lone pairs), around a central atom repel each other and arrange themselves to minimize this repulsion. This arrangement dictates the overall geometry of the molecule.
Understanding Electron Domains
The first step in applying VSEPR theory is to identify the number of electron domains around the central atom. An electron domain is a region of space where electrons are likely to be found. This includes both bonding pairs (electrons shared between atoms) and lone pairs (electrons not involved in bonding).
Example: In methane (CH₄), the central carbon atom has four bonding pairs, resulting in four electron domains. In water (H₂O), the central oxygen atom has two bonding pairs and two lone pairs, also resulting in four electron domains.
Predicting Molecular Shapes Based on Electron Domains
The number of electron domains dictates the electron-domain geometry, while the number of bonding pairs dictates the molecular geometry. Here's a summary of common geometries:
Number of Electron Domains | Electron-Domain Geometry | Molecular Geometry (Examples) | Bond Angles |
---|---|---|---|
2 | Linear | BeCl₂, CO₂ | 180° |
3 | Trigonal Planar | BF₃, SO₃ | 120° |
4 | Tetrahedral | CH₄, SiCl₄ | 109.5° |
4 (with 1 lone pair) | Tetrahedral | NH₃ | ~107° |
4 (with 2 lone pairs) | Tetrahedral | H₂O | ~104.5° |
5 | Trigonal Bipyramidal | PCl₅ | 90°, 120° |
5 (with 1 lone pair) | Trigonal Bipyramidal | SF₄ | <90°, <120° |
5 (with 2 lone pairs) | Trigonal Bipyramidal | ClF₃ | <90°, <120° |
6 | Octahedral | SF₆ | 90° |
6 (with 1 lone pair) | Octahedral | BrF₅ | <90° |
6 (with 2 lone pairs) | Octahedral | XeF₄ | 90° |
Note: The bond angles listed are ideal angles. Lone pairs exert greater repulsion than bonding pairs, leading to slightly smaller bond angles in molecules with lone pairs.
Applying VSEPR Theory: Step-by-Step Examples
Let's walk through some examples to illustrate the application of VSEPR theory:
Example 1: Carbon Dioxide (CO₂)
- Central atom: Carbon (C)
- Surrounding atoms: Two Oxygen (O) atoms
- Electron domains: Each double bond between C and O counts as one electron domain, resulting in two electron domains.
- Electron-domain geometry: Linear
- Molecular geometry: Linear
- Bond angle: 180°
Example 2: Ammonia (NH₃)
- Central atom: Nitrogen (N)
- Surrounding atoms: Three Hydrogen (H) atoms
- Electron domains: Three bonding pairs and one lone pair, resulting in four electron domains.
- Electron-domain geometry: Tetrahedral
- Molecular geometry: Trigonal pyramidal
- Bond angle: Approximately 107° (less than 109.5° due to lone pair repulsion)
Example 3: Sulfur Hexafluoride (SF₆)
- Central atom: Sulfur (S)
- Surrounding atoms: Six Fluorine (F) atoms
- Electron domains: Six bonding pairs, resulting in six electron domains.
- Electron-domain geometry: Octahedral
- Molecular geometry: Octahedral
- Bond angle: 90°
Beyond VSEPR: Hybridization and Molecular Orbital Theory
While VSEPR theory provides a simple and effective way to predict molecular shapes, it doesn't explain the bonding mechanism completely. Hybridization and molecular orbital theory offer a more detailed understanding:
Hybridization
Hybridization describes the mixing of atomic orbitals to form new hybrid orbitals that are more suitable for bonding. The type of hybridization depends on the number of electron domains:
- sp: Two electron domains (linear)
- sp²: Three electron domains (trigonal planar)
- sp³: Four electron domains (tetrahedral)
- sp³d: Five electron domains (trigonal bipyramidal)
- sp³d²: Six electron domains (octahedral)
Molecular Orbital Theory
Molecular orbital theory provides a more sophisticated description of bonding, considering the combination of atomic orbitals to form molecular orbitals that encompass the entire molecule. This theory accounts for the energy levels of the molecular orbitals and explains phenomena like bond order and magnetic properties.
Factors Influencing Molecular Shape: Exceptions to VSEPR
While VSEPR theory is remarkably accurate, there are exceptions. Certain factors can influence molecular shape, leading to deviations from the predicted geometries:
- Steric effects: Bulky substituents can cause steric hindrance, leading to distortions in bond angles.
- Multiple bonds: Multiple bonds (double and triple bonds) occupy more space than single bonds, influencing the arrangement of electron domains.
- Hyperconjugation: Interaction between sigma and pi orbitals can influence the shape and stability of the molecule.
Advanced Techniques for Determining Molecular Shape
Several advanced techniques can experimentally determine molecular shapes:
- X-ray crystallography: This technique uses X-rays to diffract off the electrons in a crystal, providing a detailed three-dimensional structure.
- Electron diffraction: Similar to X-ray crystallography but uses electrons instead of X-rays.
- Neutron diffraction: This technique utilizes neutrons to determine the positions of atoms, especially useful for locating hydrogen atoms.
- Microwave spectroscopy: This technique measures the rotational energy levels of molecules, providing information on bond lengths and angles.
The Importance of Molecular Shape in Chemistry and Beyond
Understanding molecular shapes is fundamental to many areas of chemistry and related fields:
- Reactivity: Molecular shape directly influences the accessibility of reactive sites, impacting reaction rates and mechanisms.
- Spectroscopy: Molecular shape affects the absorption and emission of electromagnetic radiation, which is crucial in spectroscopic techniques.
- Pharmacology: The shape of drug molecules is critical for their interaction with biological receptors, determining their efficacy and side effects.
- Materials science: Molecular shape plays a crucial role in the properties of materials, influencing their mechanical strength, electrical conductivity, and other characteristics.
Conclusion
Choosing the correct shape for each molecule is a fundamental skill in chemistry. VSEPR theory provides a powerful framework for predicting molecular shapes, but understanding hybridization, molecular orbital theory, and the potential influence of other factors is essential for a comprehensive understanding. Advanced experimental techniques further validate and refine our knowledge of molecular structures, highlighting the importance of molecular shape in various scientific disciplines. By mastering these concepts, you will gain a deeper understanding of the molecular world and its myriad applications.
Latest Posts
Latest Posts
-
87 5 Kg In Stones And Pounds
May 21, 2025
-
88 60 Kg In Stones And Pounds
May 21, 2025
-
How Many Oz In A Stone
May 21, 2025
-
How Many Pounds Are In 30 Kg
May 21, 2025
-
How Far Is 200 Km In Miles
May 21, 2025
Related Post
Thank you for visiting our website which covers about Choose The Correct Shape For Each Molecule . We hope the information provided has been useful to you. Feel free to contact us if you have any questions or need further assistance. See you next time and don't miss to bookmark.