Bioflix Activity How Neurons Work Action Potential Events
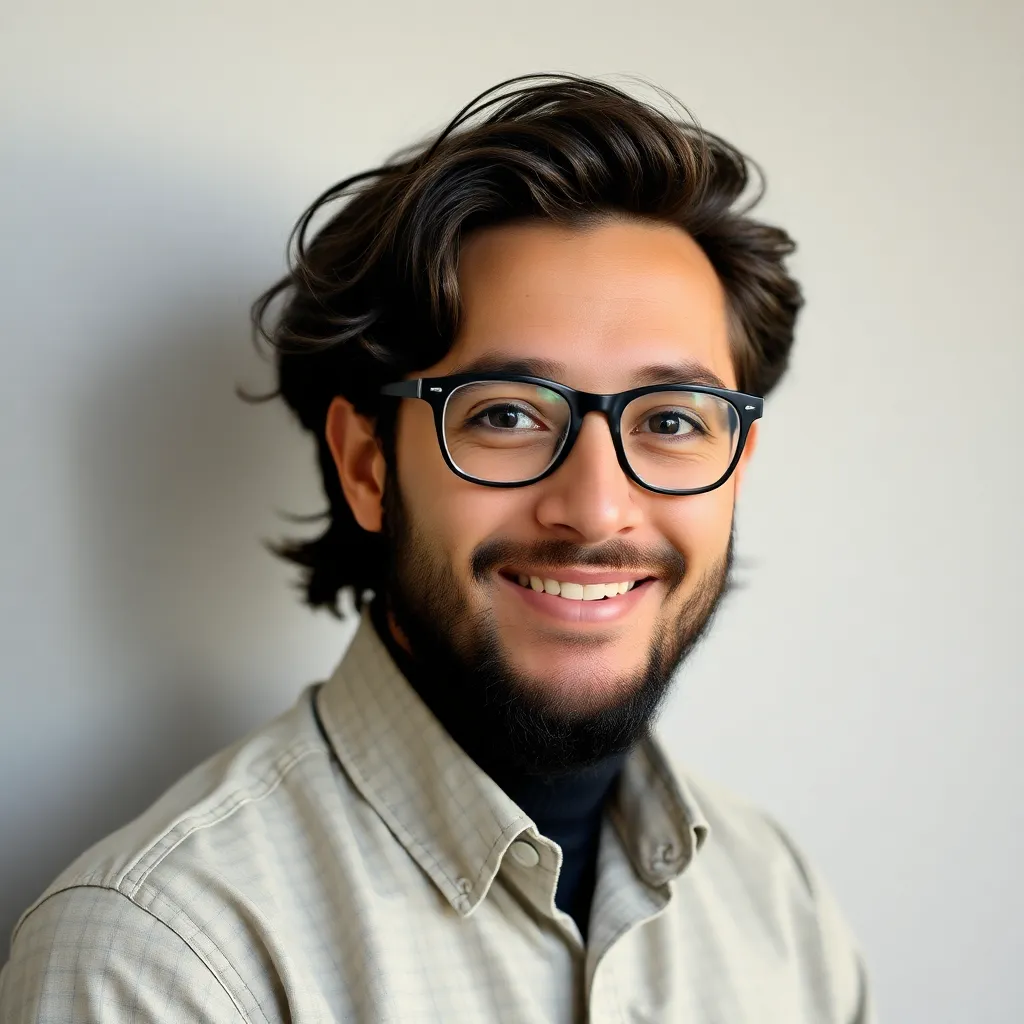
Holbox
May 12, 2025 · 6 min read
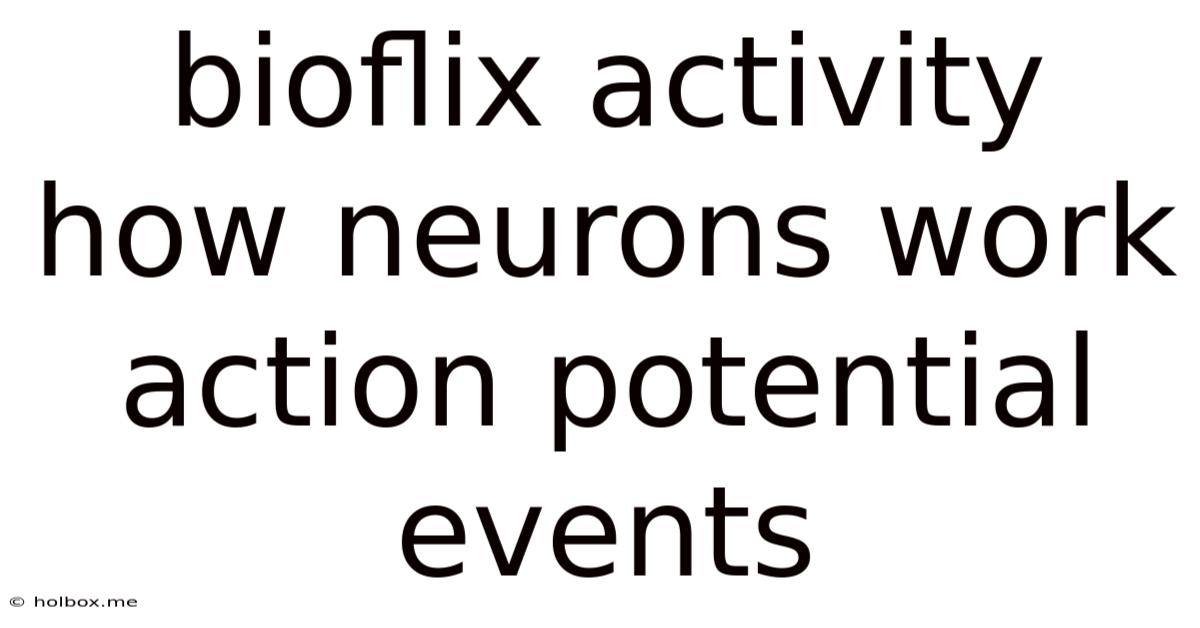
Table of Contents
- Bioflix Activity How Neurons Work Action Potential Events
- Table of Contents
- BioFlix Activity: How Neurons Work & Action Potential Events: A Deep Dive
- What are Neurons? The Fundamental Units of the Nervous System
- 1. Dendrites:
- 2. Soma (Cell Body):
- 3. Axon:
- 4. Axon Terminals (Synaptic Terminals):
- Action Potential: The Electrical Signal of Neurons
- Stages of an Action Potential (as visualized in BioFlix):
- 1. Resting Membrane Potential:
- 2. Depolarization:
- 3. Peak of Action Potential:
- 4. Repolarization:
- 5. Hyperpolarization:
- 6. Return to Resting Membrane Potential:
- The Role of Ion Channels in Action Potentials
- Propagation of Action Potentials
- Synaptic Transmission: Communication Between Neurons
- Clinical Implications and Research
- Conclusion: BioFlix and the Future of Neuroscience Education
- Latest Posts
- Related Post
BioFlix Activity: How Neurons Work & Action Potential Events: A Deep Dive
Understanding how neurons work is fundamental to comprehending the complexities of the nervous system. This article delves into the intricacies of neuronal function, focusing on action potentials and using the BioFlix activity as a framework for understanding these critical events. We’ll explore the key players involved, the steps of action potential generation, and the significance of these processes in overall neurological function. We'll also touch upon relevant clinical implications and future research directions.
What are Neurons? The Fundamental Units of the Nervous System
Neurons are specialized cells that transmit information throughout the body via electrical and chemical signals. They are the building blocks of the brain, spinal cord, and peripheral nervous system, responsible for everything from simple reflexes to complex cognitive processes. A typical neuron consists of several key components:
1. Dendrites:
These branching extensions receive signals from other neurons. Think of them as the neuron's "input" zone. Neurotransmitters released from neighboring neurons bind to receptors on the dendrites, initiating electrical changes.
2. Soma (Cell Body):
The soma contains the neuron's nucleus and other organelles responsible for cell maintenance and function. It integrates the incoming signals from the dendrites.
3. Axon:
The axon is a long, slender projection that transmits signals away from the soma. This is the "output" zone. The axon's length varies greatly, ranging from a few micrometers to over a meter.
4. Axon Terminals (Synaptic Terminals):
These are the branched endings of the axon that form connections (synapses) with other neurons or target cells (e.g., muscle cells). They release neurotransmitters to communicate with these target cells.
Action Potential: The Electrical Signal of Neurons
The action potential is a rapid, transient change in the electrical potential across the neuronal membrane. This is the fundamental way neurons transmit information over long distances. It's an all-or-nothing event: either an action potential occurs, or it doesn't. The strength of a stimulus does not affect the amplitude of the action potential; instead, it affects the frequency of action potentials.
Stages of an Action Potential (as visualized in BioFlix):
The BioFlix activity provides an excellent visual representation of the action potential's stages. Let's break them down:
1. Resting Membrane Potential:
Before an action potential, the neuron is at its resting membrane potential, typically around -70 mV. This negative potential is maintained by the uneven distribution of ions (sodium (Na+), potassium (K+), chloride (Cl-), and negatively charged proteins) across the neuronal membrane. The sodium-potassium pump actively transports sodium ions out of the cell and potassium ions into the cell, contributing significantly to this resting potential.
2. Depolarization:
A stimulus (e.g., neurotransmitter binding to a receptor) causes the membrane potential to become less negative. If the stimulus is strong enough to reach the threshold potential (typically around -55 mV), voltage-gated sodium channels open. Sodium ions rush into the cell, causing a rapid depolarization – a significant positive shift in membrane potential.
3. Peak of Action Potential:
The membrane potential reaches its peak, typically around +30 mV. At this point, sodium channels begin to inactivate, and potassium channels begin to open.
4. Repolarization:
Potassium ions flow out of the cell, making the membrane potential more negative again. This process is called repolarization.
5. Hyperpolarization:
The membrane potential briefly becomes more negative than the resting membrane potential (hyperpolarization). This is due to the continued outflow of potassium ions and the slow closure of potassium channels.
6. Return to Resting Membrane Potential:
The sodium-potassium pump and other ion channels restore the original ion distribution, bringing the membrane potential back to its resting state. The neuron is now ready to fire another action potential.
The Role of Ion Channels in Action Potentials
The action potential relies heavily on the precise functioning of voltage-gated ion channels. These channels are proteins embedded in the neuronal membrane that open and close in response to changes in the membrane potential.
- Voltage-gated sodium channels: Responsible for the rapid influx of sodium ions during depolarization.
- Voltage-gated potassium channels: Responsible for the outflow of potassium ions during repolarization.
The precise timing and kinetics of these channels are crucial for the shape and speed of the action potential. Disruptions in these channels can lead to neurological disorders.
Propagation of Action Potentials
Once an action potential is initiated, it propagates along the axon without decrement. This means the signal doesn't weaken as it travels. The process involves a chain reaction of depolarization and repolarization events along the axon's length. In myelinated axons (axons covered with myelin sheath), the action potential jumps between the Nodes of Ranvier (gaps in the myelin sheath), a process called saltatory conduction. This significantly increases the speed of conduction.
Synaptic Transmission: Communication Between Neurons
Once the action potential reaches the axon terminals, it triggers the release of neurotransmitters into the synaptic cleft, the space between the presynaptic neuron and the postsynaptic neuron. These neurotransmitters bind to receptors on the postsynaptic neuron, initiating either excitatory postsynaptic potentials (EPSPs) or inhibitory postsynaptic potentials (IPSPs).
- EPSPs: Depolarize the postsynaptic neuron, making it more likely to fire an action potential.
- IPSPs: Hyperpolarize the postsynaptic neuron, making it less likely to fire an action potential.
The integration of EPSPs and IPSPs determines whether the postsynaptic neuron will fire an action potential.
Clinical Implications and Research
Understanding action potentials is crucial for understanding many neurological disorders. Disruptions in ion channel function, neurotransmitter release, or synaptic transmission can lead to a wide range of conditions, including:
- Epilepsy: Characterized by abnormal neuronal activity and seizures.
- Multiple sclerosis (MS): An autoimmune disease that damages the myelin sheath, disrupting action potential propagation.
- Alzheimer's disease: A neurodegenerative disease associated with impaired synaptic transmission.
- Parkinson's disease: A neurodegenerative disease involving the loss of dopaminergic neurons.
Ongoing research continues to explore the intricate mechanisms of action potentials and their role in health and disease. This research is critical for developing new treatments and therapies for neurological disorders. Advances in imaging techniques, genetic engineering, and computational modeling are providing valuable insights into neuronal function at the molecular, cellular, and systems levels. Future research will likely focus on refining our understanding of how complex neural circuits process information and on developing more effective strategies for repairing damaged neural pathways.
Conclusion: BioFlix and the Future of Neuroscience Education
The BioFlix activity provides a powerful tool for visualizing the complex processes involved in neuronal function and action potential generation. By providing an interactive and engaging learning experience, it helps students and researchers alike gain a deeper understanding of these fundamental mechanisms. As technology continues to advance, we can expect even more sophisticated tools and techniques to emerge, further enhancing our understanding of the nervous system and its intricate workings. The continued exploration of neuronal function will undoubtedly lead to breakthroughs in the diagnosis, treatment, and prevention of neurological disorders, ultimately improving human health and well-being. The detailed and interactive nature of the BioFlix simulations allows for a more comprehensive grasp of these complex processes, bridging the gap between abstract concepts and concrete understanding. This makes it an invaluable resource for anyone seeking to enhance their knowledge of neuroscience.
Latest Posts
Related Post
Thank you for visiting our website which covers about Bioflix Activity How Neurons Work Action Potential Events . We hope the information provided has been useful to you. Feel free to contact us if you have any questions or need further assistance. See you next time and don't miss to bookmark.