Art-labeling Activity The Structures Of Electrical And Chemical Synapses
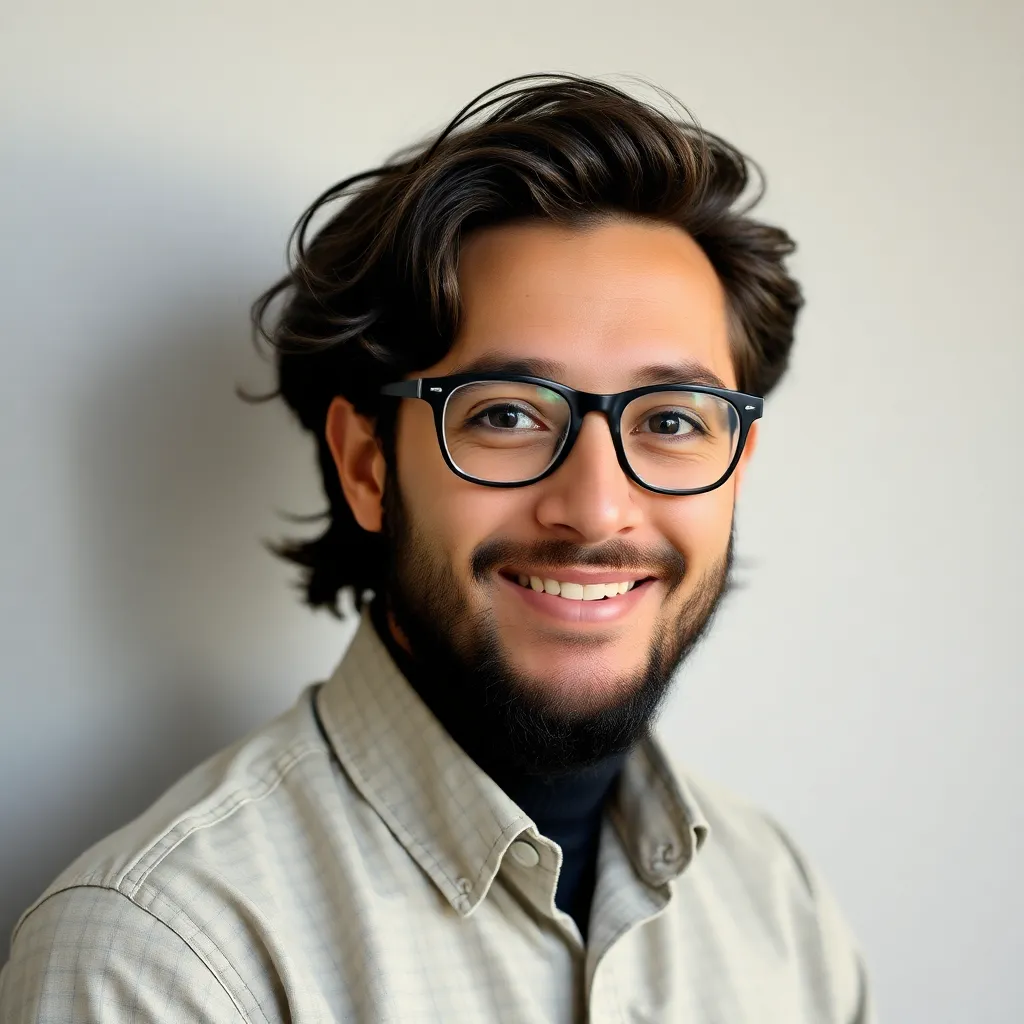
Holbox
Apr 25, 2025 · 6 min read
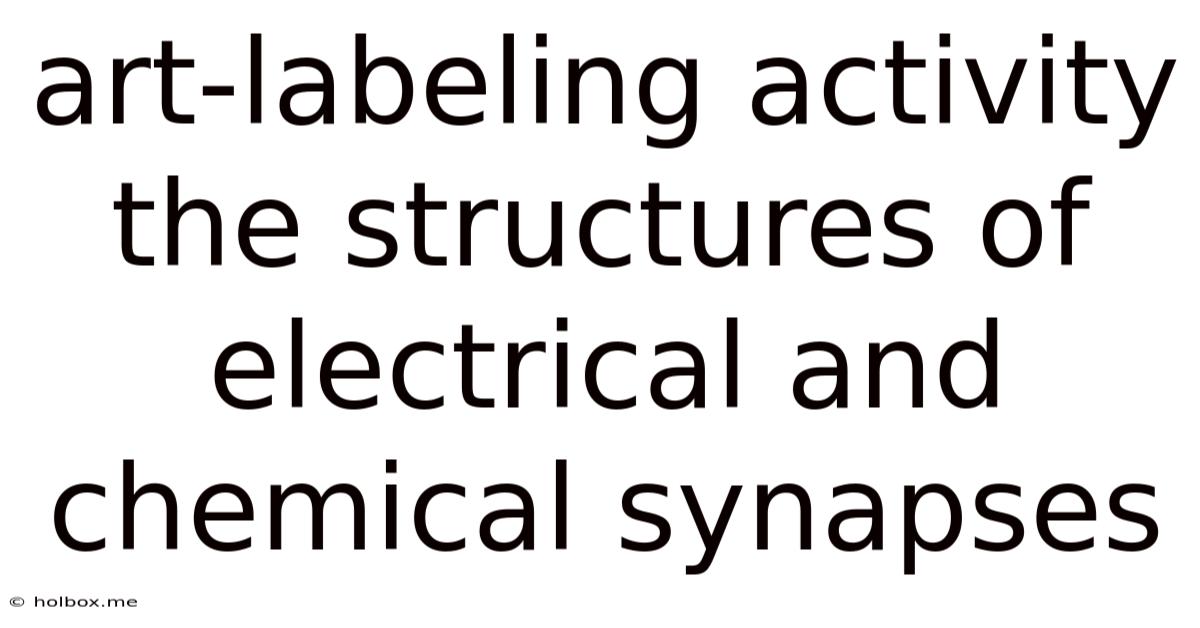
Table of Contents
- Art-labeling Activity The Structures Of Electrical And Chemical Synapses
- Table of Contents
- Art-Labeling Activity: Unveiling the Structures of Electrical and Chemical Synapses
- I. The Electrifying Speed of Electrical Synapses: A Structural Overview
- A. Gap Junctions: The Architectural Marvels of Electrical Synapses
- B. The Cytoplasmic Bridge: Direct Electrical Coupling
- C. Art-Labeling the Electrical Synapse: A Summary
- II. The Chemical Symphony: Deconstructing the Structure of Chemical Synapses
- A. The Presynaptic Terminal: The Message Composer
- B. The Synaptic Cleft: The Communication Bridge
- C. The Postsynaptic Density: The Message Receiver
- D. Art-Labeling the Chemical Synapse: A Comprehensive Depiction
- III. Comparative Analysis: Electrical vs. Chemical Synapses - A Structured Comparison
- IV. Beyond the Basics: The Dynamic Nature of Synapses
- V. Conclusion: An Artist's Impression of Synaptic Communication
- Latest Posts
- Latest Posts
- Related Post
Art-Labeling Activity: Unveiling the Structures of Electrical and Chemical Synapses
The intricate world of neural communication hinges on the precise interactions between neurons at synapses. Understanding the structures of these connections – electrical and chemical synapses – is crucial to comprehending brain function, neurological disorders, and the potential for therapeutic interventions. This article will delve into the detailed structures of both types of synapses, employing an "art-labeling" approach to enhance comprehension. Imagine each description as a meticulously crafted artwork, where each labeled element contributes to the overall masterpiece of synaptic transmission.
I. The Electrifying Speed of Electrical Synapses: A Structural Overview
Electrical synapses, characterized by their rapid transmission of signals, achieve this speed through direct cytoplasmic connections between neurons. These connections are formed by gap junctions, the stars of our "art piece."
A. Gap Junctions: The Architectural Marvels of Electrical Synapses
Gap junctions are clusters of connexons, each a hexamer of connexin proteins. These connexins are transmembrane proteins, meaning they span the entire neuronal membrane. Think of them as tiny, meticulously crafted tunnels, perfectly aligned between pre- and postsynaptic neurons. Each connexon from one neuron aligns perfectly with a connexon from the neighboring neuron, forming a continuous channel.
- Connexon Structure: Imagine a six-pointed star, each point representing a single connexin protein. These proteins are arranged in a precise manner, creating a central pore – the conduit for electrical signal transmission.
- Channel Diameter and Selectivity: This central pore has a diameter that allows the passage of small ions and molecules, such as sodium (Na+), potassium (K+), and calcium (Ca2+), but restricts the passage of larger molecules. This selectivity is a critical element in regulating the flow of information.
- Bidirectional Transmission: Unlike chemical synapses, electrical synapses allow for bidirectional transmission of signals. This means that the flow of ions can occur in both directions – from the presynaptic neuron to the postsynaptic neuron and vice versa. This characteristic is essential in certain neural circuits requiring rapid, synchronized activity, such as those involved in escape reflexes.
B. The Cytoplasmic Bridge: Direct Electrical Coupling
The gap junction provides a direct cytoplasmic connection between pre- and postsynaptic neurons. This is unlike chemical synapses where a synaptic cleft separates the neurons. The close proximity allows for near-instantaneous transmission of the electrical signal. Visualize this as a direct, seamless bridge between two neighboring houses, allowing for the immediate passage of information.
- Low Resistance Pathway: The gap junction creates a pathway with extremely low electrical resistance, minimizing signal loss during transmission.
- Synchronization: This low resistance and direct coupling enables synchronization of neuronal activity within a network. This synchronized activity is crucial for many brain functions, including rhythmic activities like respiration and locomotion.
C. Art-Labeling the Electrical Synapse: A Summary
Our "art piece" of the electrical synapse showcases the beautifully aligned connexons forming gap junctions, creating a direct, low-resistance pathway between the pre- and postsynaptic neurons. The labels would highlight the connexins, connexons, the gap junction itself, and the minimal synaptic cleft, emphasizing the direct cytoplasmic continuity.
II. The Chemical Symphony: Deconstructing the Structure of Chemical Synapses
Chemical synapses, far more prevalent than electrical synapses, use neurotransmitters to transmit signals across a synaptic cleft. This process is more complex and slower but allows for greater flexibility and modulation of synaptic transmission.
A. The Presynaptic Terminal: The Message Composer
The presynaptic terminal, also known as the axon terminal, is where the action begins. Here, we find an array of crucial structures responsible for neurotransmitter synthesis, packaging, and release.
- Synaptic Vesicles: These small membrane-bound sacs are like tiny parcels containing neurotransmitters. They are strategically positioned near the presynaptic membrane, awaiting release.
- Active Zones: These specialized regions on the presynaptic membrane are where synaptic vesicles fuse to release their neurotransmitter cargo. Imagine these as carefully designated docking stations.
- Voltage-Gated Calcium Channels: These channels are vital for neurotransmitter release. Upon arrival of an action potential, they open, allowing an influx of calcium ions (Ca2+) into the presynaptic terminal. The increased Ca2+ concentration triggers the fusion of synaptic vesicles with the presynaptic membrane.
B. The Synaptic Cleft: The Communication Bridge
The synaptic cleft, a fluid-filled space separating the pre- and postsynaptic neurons, is the central feature of our "chemical synapse artwork." It’s a crucial component that differentiates chemical synapses from electrical synapses.
- Neurotransmitter Diffusion: Released neurotransmitters diffuse across this cleft, reaching the postsynaptic membrane. This diffusion is a critical step, influencing the speed and effectiveness of synaptic transmission.
- Extracellular Matrix: The synaptic cleft isn't just empty space; it contains an extracellular matrix that provides structural support and may also influence neurotransmitter diffusion and degradation.
C. The Postsynaptic Density: The Message Receiver
The postsynaptic density (PSD) is a highly organized protein complex on the postsynaptic membrane. It's a bustling hub of activity, responsible for receiving and processing the neurotransmitter signal.
- Neurotransmitter Receptors: These specialized receptors bind to specific neurotransmitters, initiating a cascade of events within the postsynaptic neuron. Think of them as precisely engineered locks, accepting only the correct neurotransmitter "keys."
- Signal Transduction Pathways: Binding of neurotransmitters to their receptors activates intracellular signaling pathways, leading to changes in postsynaptic membrane potential or other cellular processes. This is the complex choreography of signal transduction, where multiple proteins interact to relay the signal.
- Scaffolding Proteins: These proteins provide structural support and organize the various components of the PSD. They are like the scaffolding of a building, ensuring the stability and correct positioning of all the components.
D. Art-Labeling the Chemical Synapse: A Comprehensive Depiction
Our "artwork" of the chemical synapse would showcase the presynaptic terminal with its synaptic vesicles clustered near the active zones, voltage-gated calcium channels prominently displayed. The synaptic cleft would be clearly defined, and the postsynaptic density would be meticulously labeled, highlighting the neurotransmitter receptors, signal transduction proteins, and scaffolding proteins.
III. Comparative Analysis: Electrical vs. Chemical Synapses - A Structured Comparison
Feature | Electrical Synapse | Chemical Synapse |
---|---|---|
Transmission | Direct, electrical coupling via gap junctions | Indirect, chemical signaling via neurotransmitters |
Speed | Very fast, near instantaneous | Relatively slow |
Direction | Bidirectional | Unidirectional (generally) |
Signal Strength | Relatively constant | Highly variable, subject to modulation |
Synaptic Cleft | Minimal or absent | Significant cleft present |
Plasticity | Low plasticity | High plasticity (synaptic strength can be modified) |
Types of Signals | Primarily excitatory | Excitatory or inhibitory |
IV. Beyond the Basics: The Dynamic Nature of Synapses
The structures described above are not static; synapses are highly dynamic entities. Their structures and functions can be modified by various factors, including:
- Synaptic Plasticity: The ability of synapses to strengthen or weaken over time, a crucial mechanism underlying learning and memory.
- Neurotrophic Factors: Proteins that influence synapse development, maintenance, and function.
- Disease: Many neurological disorders are associated with alterations in synaptic structure and function. Understanding these changes is crucial for developing effective therapies.
V. Conclusion: An Artist's Impression of Synaptic Communication
This "art-labeling" approach has provided a detailed and hopefully engaging exploration of electrical and chemical synapses. Understanding the intricate architecture of these structures is essential for comprehending the complexity of neural communication. The dynamic interplay between these different types of synapses orchestrates the symphony of brain function, shaping our thoughts, actions, and experiences. Further research into the intricacies of synaptic structures and their plasticity promises to unravel more secrets of the brain, paving the way for advancements in the diagnosis and treatment of neurological disorders. The journey of unveiling the mysteries of the synapse is an ongoing artistic process, with new details continually being revealed.
Latest Posts
Latest Posts
-
Myofilament With A Knob Like Head
May 09, 2025
-
If The Financial Markets Are Efficient Then
May 09, 2025
-
Medical Terminology An Illustrated Guide Ninth Edition
May 09, 2025
-
One Way To Overcome The Principal Agent Problem Is To
May 09, 2025
-
Certain Noteheads Can Only Be Placed On Staff Lines
May 09, 2025
Related Post
Thank you for visiting our website which covers about Art-labeling Activity The Structures Of Electrical And Chemical Synapses . We hope the information provided has been useful to you. Feel free to contact us if you have any questions or need further assistance. See you next time and don't miss to bookmark.