An Action Potential Is Self-regenerating Because __________.
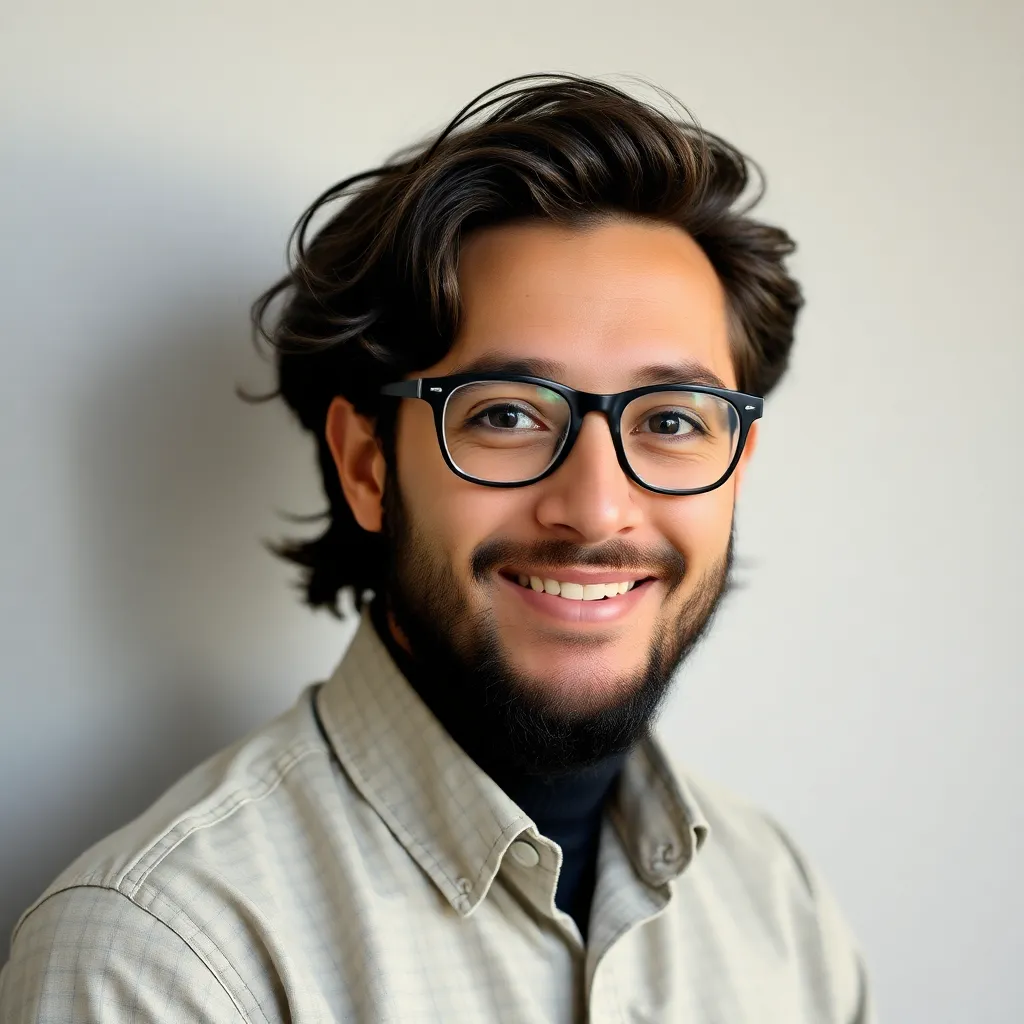
Holbox
Apr 16, 2025 · 7 min read
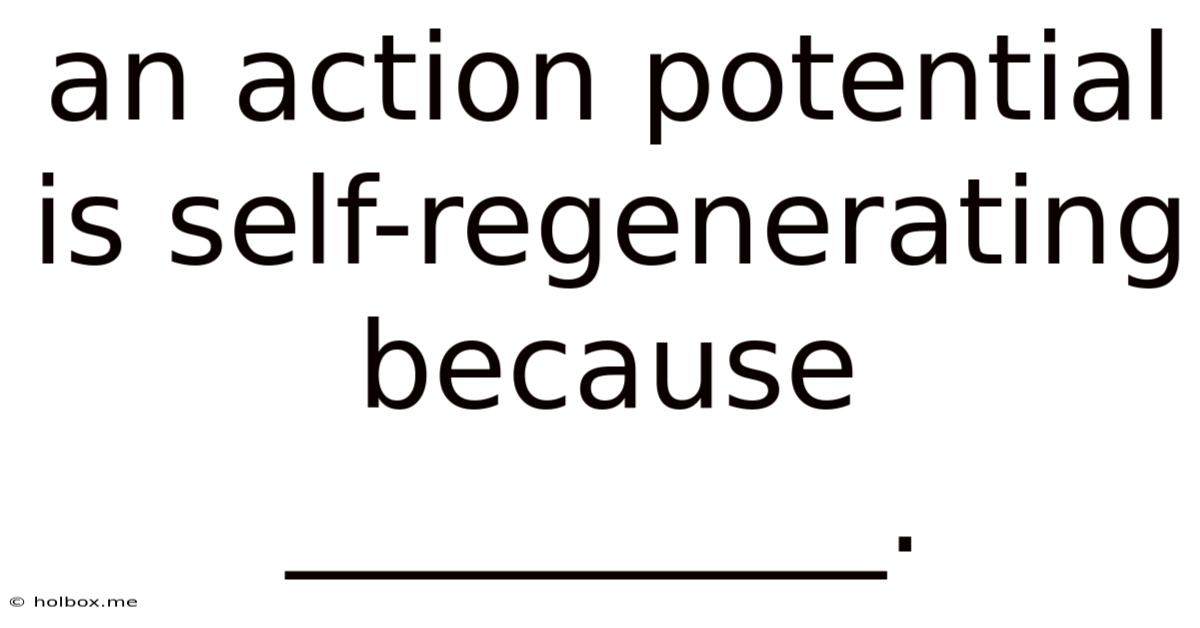
Table of Contents
- An Action Potential Is Self-regenerating Because __________.
- Table of Contents
- An Action Potential is Self-Regenerating Because of Positive Feedback and Ion Channel Dynamics
- The All-or-None Principle and the Positive Feedback Loop
- Understanding the Positive Feedback Mechanism
- The Role of Voltage-Gated Potassium Channels (K⁺)
- Repolarization and Refractoriness
- Spatial and Temporal Dynamics: How the Signal Propagates
- Local Circuit Currents and Depolarization
- Myelin Sheath and Saltatory Conduction
- Ion Channel Properties and Their Contribution to Self-Regeneration
- Voltage-Sensitivity and Activation/Inactivation Kinetics
- Channel Density and Distribution
- Variations in Ion Channel Properties Across Neuron Types
- The Significance of Self-Regeneration in Neuronal Signaling
- Long-Distance Signaling
- Reliable Signal Transmission
- Precise Temporal Coding
- Conclusion
- Latest Posts
- Latest Posts
- Related Post
An Action Potential is Self-Regenerating Because of Positive Feedback and Ion Channel Dynamics
An action potential, the fundamental unit of neuronal signaling, is a remarkable phenomenon. Its ability to rapidly propagate along the axon without decrement – its self-regenerating nature – is crucial for efficient communication within the nervous system. This self-regeneration isn't a passive process; it's actively driven by a complex interplay of positive feedback loops and the precise temporal dynamics of voltage-gated ion channels. Let's delve into the mechanisms that underpin this essential characteristic.
The All-or-None Principle and the Positive Feedback Loop
The self-regenerating nature of an action potential is intimately tied to the all-or-none principle. This principle states that an action potential either occurs completely or not at all; there are no "partial" action potentials. This decisive response stems from a positive feedback loop involving voltage-gated sodium (Na⁺) channels.
Understanding the Positive Feedback Mechanism
-
Depolarization: When a neuron receives sufficient excitatory input, its membrane potential depolarizes, reaching a critical threshold potential.
-
Sodium Channel Activation: This depolarization triggers the opening of voltage-gated Na⁺ channels. These channels are sensitive to changes in membrane potential; as the membrane depolarizes, more channels open.
-
Sodium Influx: The opening of Na⁺ channels allows a rapid influx of Na⁺ ions into the neuron, further depolarizing the membrane potential.
-
Positive Feedback: This further depolarization opens even more Na⁺ channels, leading to a greater influx of Na⁺ ions. This creates a positive feedback loop: depolarization → channel opening → more depolarization → more channel opening, and so on.
-
Peak Potential: This positive feedback loop continues until the membrane potential reaches its peak, typically around +30 mV. At this point, Na⁺ channel inactivation begins.
The crucial point is that the initial depolarization, even a relatively small one above threshold, triggers a cascade of events that leads to a full-blown action potential. This is the self-regenerating aspect: the initial stimulus isn't merely propagated; it's amplified. The positive feedback loop ensures that the signal doesn't weaken as it travels down the axon.
The Role of Voltage-Gated Potassium Channels (K⁺)
While the voltage-gated Na⁺ channels are responsible for the rapid depolarization phase of the action potential, the voltage-gated potassium (K⁺) channels play a crucial role in repolarization and refractoriness, ensuring the unidirectional propagation of the action potential.
Repolarization and Refractoriness
-
Potassium Channel Activation: As the membrane potential reaches its peak, voltage-gated K⁺ channels begin to open.
-
Potassium Efflux: This opening allows the efflux of K⁺ ions out of the neuron, causing the membrane potential to repolarize.
-
Hyperpolarization: The outflow of K⁺ ions often leads to a transient hyperpolarization, making the membrane potential more negative than the resting potential. This hyperpolarization contributes to the refractory period.
-
Refractory Period: The refractory period is a crucial aspect of action potential propagation. It is the time during which another action potential cannot be generated in the same section of the axon. This is primarily due to the inactivation of Na⁺ channels and the continued outward current of K⁺ ions.
The refractory period ensures that the action potential travels in only one direction, preventing backward propagation. Once the membrane potential is restored to the resting level, the neuron is again capable of generating another action potential.
Spatial and Temporal Dynamics: How the Signal Propagates
The self-regenerating nature of the action potential allows it to propagate effectively along the length of the axon. This propagation relies on the spatial and temporal dynamics of ion channel opening and closing.
Local Circuit Currents and Depolarization
-
Initial Depolarization: The initial depolarization at the axon hillock (the region where the axon originates from the cell body) triggers the opening of voltage-gated Na⁺ channels in that area.
-
Local Circuit Currents: The influx of Na⁺ ions creates a localized region of high positive charge. This creates an electrical current, a local circuit current, that flows passively along the axon, depolarizing adjacent membrane regions.
-
Threshold Exceeded: If the depolarization caused by the local circuit current reaches the threshold potential in the adjacent region, it triggers the opening of voltage-gated Na⁺ channels in that area, initiating a new action potential.
-
Sequential Depolarization: This process repeats itself sequentially along the axon, with each action potential triggering the next in a chain reaction. This ensures that the signal travels the entire length of the axon without decrement.
Myelin Sheath and Saltatory Conduction
In myelinated axons, the propagation of action potentials is significantly faster due to saltatory conduction. Myelin, a fatty insulating sheath, prevents ion flow across the axon membrane except at the Nodes of Ranvier.
-
Nodes of Ranvier: These nodes are gaps in the myelin sheath where voltage-gated ion channels are concentrated.
-
Jumping of the Signal: The action potential "jumps" from one Node of Ranvier to the next, effectively bypassing the myelinated segments. This "jumping" increases the speed of conduction dramatically.
The saltatory conduction further emphasizes the self-regenerating nature of the action potential. The signal isn't merely passively conducted; it's repeatedly regenerated at each Node of Ranvier, ensuring its strength remains constant even over long distances.
Ion Channel Properties and Their Contribution to Self-Regeneration
The specific properties of the voltage-gated Na⁺ and K⁺ channels are critically important for the self-regenerating nature of the action potential.
Voltage-Sensitivity and Activation/Inactivation Kinetics
The voltage sensitivity of these channels, meaning their propensity to open or close at specific membrane potentials, is precisely tuned. The rapid activation of Na⁺ channels at threshold and their subsequent inactivation, coupled with the slower activation of K⁺ channels, are essential for the characteristic shape and propagation of the action potential.
Channel Density and Distribution
The density and distribution of these channels along the axon also contribute to self-regeneration. The high density of Na⁺ channels at the axon hillock ensures efficient initiation of the action potential, while their distribution along the axon (or at the Nodes of Ranvier in myelinated axons) ensures its propagation.
Variations in Ion Channel Properties Across Neuron Types
It's important to remember that the properties of voltage-gated ion channels can vary across different neuron types. This variation contributes to the diversity of action potential characteristics observed in different parts of the nervous system. These differences in channel properties reflect the specific functional requirements of different neurons. For instance, some neurons require faster conduction speeds than others, influencing the density and distribution of ion channels.
The Significance of Self-Regeneration in Neuronal Signaling
The self-regenerating property of the action potential is not merely an interesting biological phenomenon; it's essential for the proper functioning of the nervous system.
Long-Distance Signaling
Without self-regeneration, the signal would weaken over long distances, rendering long-axon neurons ineffective. The ability of the action potential to propagate without decrement is essential for transmitting information across significant distances within the body.
Reliable Signal Transmission
The all-or-none nature and self-regenerating characteristics of the action potential ensure reliable transmission of signals. The signal either arrives fully formed or not at all, minimizing information loss. This is crucial for accurate processing of information in the brain and other parts of the nervous system.
Precise Temporal Coding
The precise timing of action potentials is crucial for many neural processes. The self-regenerating nature of the action potential ensures that the temporal information encoded in the pattern of action potentials is preserved during propagation. This temporal coding underlies many aspects of neural computation.
Conclusion
In summary, an action potential is self-regenerating because of the positive feedback loop involving voltage-gated sodium channels and the carefully orchestrated interplay between sodium and potassium channel dynamics. This self-regeneration, facilitated by local circuit currents and, in myelinated axons, saltatory conduction, ensures efficient, reliable, and temporally precise transmission of information throughout the nervous system. The exquisite control of ion channel properties, their density, and distribution, underscores the sophisticated biological mechanisms that underpin this fundamental process of neuronal communication. Variations in these properties across neuron types allow for adaptation to specific functional needs, highlighting the adaptability and complexity of the nervous system.
Latest Posts
Latest Posts
-
Accounts Receivable Arises When A Company
Apr 25, 2025
-
What Is The Meaning Of The Phrase Dilemma Of Regulation
Apr 25, 2025
-
How Many Parallel Sides Can A Triangle Have
Apr 25, 2025
-
Complete The Following Scheme Of Circulation In The Human Body
Apr 25, 2025
-
Identify The True Statement About Windows Tasks
Apr 25, 2025
Related Post
Thank you for visiting our website which covers about An Action Potential Is Self-regenerating Because __________. . We hope the information provided has been useful to you. Feel free to contact us if you have any questions or need further assistance. See you next time and don't miss to bookmark.