Advance Study Assignment The Geometrical Structure Of Molecules
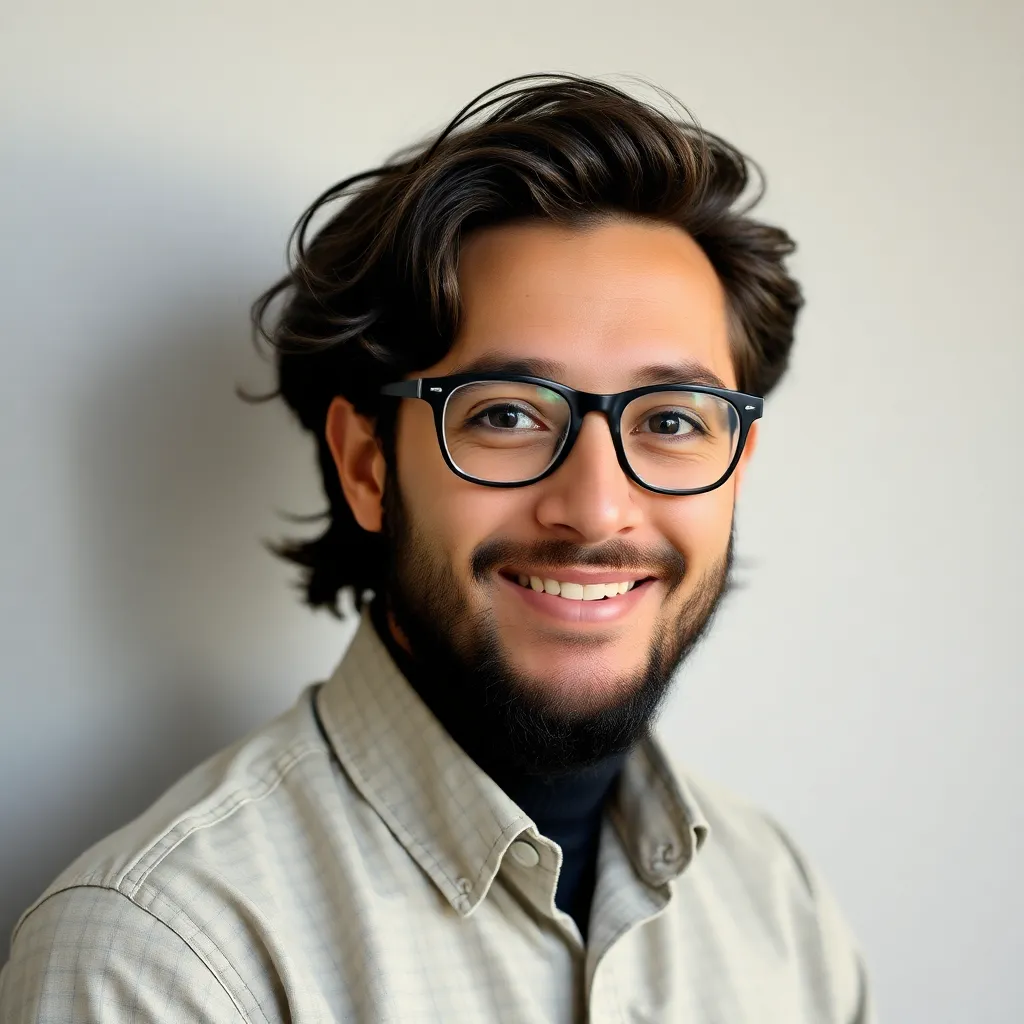
Holbox
Apr 08, 2025 · 6 min read
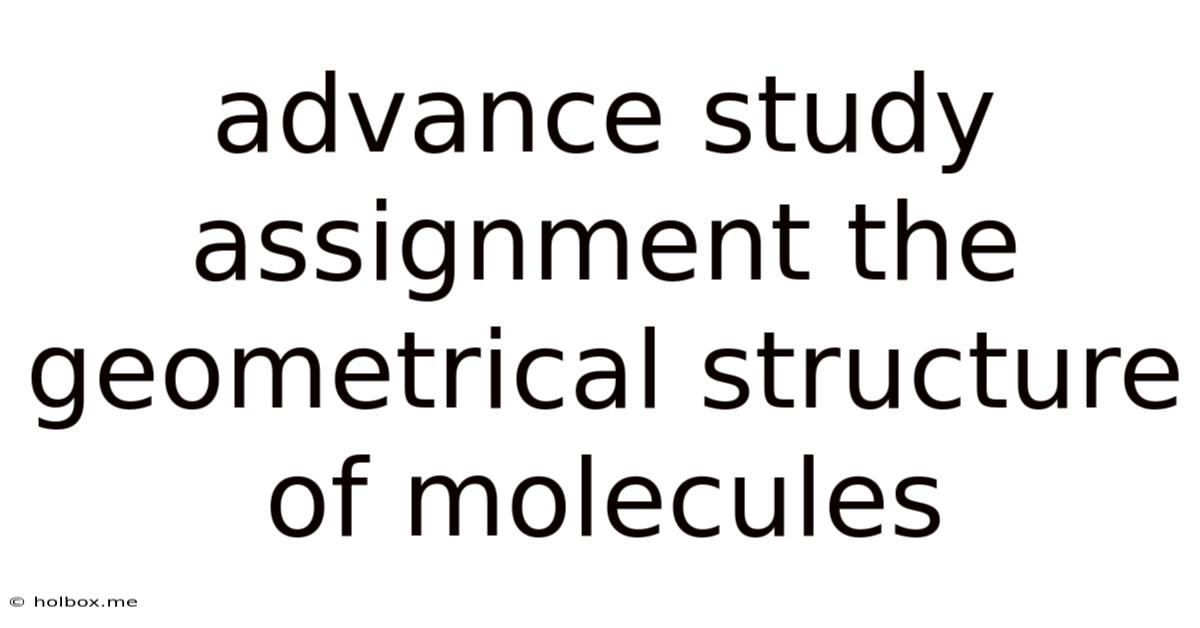
Table of Contents
- Advance Study Assignment The Geometrical Structure Of Molecules
- Table of Contents
- Advanced Study Assignment: The Geometrical Structure of Molecules
- I. The Valence Shell Electron Pair Repulsion (VSEPR) Theory
- A. Electron Domains and Geometry
- B. Lone Pairs vs. Bonding Pairs
- C. Predicting Molecular Geometry using VSEPR
- II. Valence Bond Theory and Hybridization
- A. Atomic Orbitals and Overlap
- B. Hybridization
- C. Relating Hybridization to Molecular Geometry
- III. Molecular Shapes and their Properties
- A. Polarity
- B. Boiling Point and Melting Point
- C. Reactivity
- IV. Advanced Examples and Applications
- A. SF₆ (Sulfur Hexafluoride)
- B. XeF₄ (Xenon Tetrafluoride)
- C. IF₅ (Iodine Pentafluoride)
- V. Limitations of VSEPR and Valence Bond Theory
- VI. Conclusion
- Latest Posts
- Latest Posts
- Related Post
Advanced Study Assignment: The Geometrical Structure of Molecules
Understanding the geometrical structure of molecules is fundamental to chemistry. Molecular geometry dictates a molecule's physical and chemical properties, influencing everything from its reactivity to its boiling point. This advanced study assignment delves into the principles governing molecular shape, exploring various theories and models used to predict and understand these structures. We'll examine the VSEPR theory, valence bond theory, and hybridization, and explore their applications in determining the shapes of diverse molecules.
I. The Valence Shell Electron Pair Repulsion (VSEPR) Theory
The VSEPR theory provides a simple yet powerful method for predicting molecular geometry. Its central premise is that electron pairs in the valence shell of a central atom repel each other and arrange themselves to maximize the distance between them, minimizing repulsion. This arrangement determines the overall shape of the molecule.
A. Electron Domains and Geometry
The VSEPR theory considers both bonding and non-bonding (lone) electron pairs when predicting molecular geometry. Each electron pair, whether bonding or non-bonding, occupies an electron domain. The number of electron domains around the central atom dictates the basic geometry:
- Two electron domains: Linear geometry (180° bond angle)
- Three electron domains: Trigonal planar geometry (120° bond angle)
- Four electron domains: Tetrahedral geometry (109.5° bond angle)
- Five electron domains: Trigonal bipyramidal geometry (90° and 120° bond angles)
- Six electron domains: Octahedral geometry (90° bond angle)
B. Lone Pairs vs. Bonding Pairs
It's crucial to distinguish between lone pairs and bonding pairs. Lone pairs exert a stronger repulsive force than bonding pairs due to their closer proximity to the central atom. This results in deviations from the idealized geometries. For example, a molecule with four electron domains (tetrahedral) will have a different shape if one or more of those domains are lone pairs.
- Ammonia (NH₃): Four electron domains (three bonding pairs, one lone pair). The geometry is tetrahedral, but the molecular shape (considering only the atoms) is trigonal pyramidal.
- Water (H₂O): Four electron domains (two bonding pairs, two lone pairs). The geometry is tetrahedral, but the molecular shape is bent.
C. Predicting Molecular Geometry using VSEPR
To predict the geometry of a molecule using VSEPR:
- Draw the Lewis structure: This determines the number of electron domains around the central atom.
- Determine the electron domain geometry: Based on the number of electron domains, identify the basic geometry (linear, trigonal planar, etc.).
- Consider the lone pairs: Lone pairs influence the molecular shape, causing deviations from the ideal geometry.
- Name the molecular geometry: Describe the arrangement of atoms, not including lone pairs.
II. Valence Bond Theory and Hybridization
While VSEPR effectively predicts molecular geometry, valence bond theory provides a deeper understanding of the bonding involved. It describes covalent bonds as the overlap of atomic orbitals. Hybridization extends this theory by explaining how atomic orbitals combine to form hybrid orbitals, which are more suitable for bonding.
A. Atomic Orbitals and Overlap
Atomic orbitals, such as s and p orbitals, have specific shapes and orientations. Covalent bonds form when atomic orbitals from different atoms overlap, resulting in a region of high electron density between the nuclei. The greater the overlap, the stronger the bond.
B. Hybridization
Hybridization involves the mixing of atomic orbitals to create new hybrid orbitals with different shapes and energies. The types of hybrid orbitals depend on the number of atomic orbitals involved:
- sp hybridization: One s orbital and one p orbital combine to form two sp hybrid orbitals oriented linearly (180°).
- sp² hybridization: One s orbital and two p orbitals combine to form three sp² hybrid orbitals oriented in a trigonal planar arrangement (120°).
- sp³ hybridization: One s orbital and three p orbitals combine to form four sp³ hybrid orbitals arranged tetrahedrally (109.5°).
- sp³d hybridization: One s, three p, and one d orbital combine to form five sp³d hybrid orbitals arranged in a trigonal bipyramidal geometry.
- sp³d² hybridization: One s, three p, and two d orbitals combine to form six sp³d² hybrid orbitals arranged octahedrally.
C. Relating Hybridization to Molecular Geometry
The type of hybridization adopted by the central atom directly correlates with the molecular geometry predicted by VSEPR. For instance, a molecule with sp³ hybridization will have a tetrahedral electron domain geometry, consistent with VSEPR predictions.
III. Molecular Shapes and their Properties
The geometrical structure of a molecule profoundly impacts its physical and chemical properties.
A. Polarity
Molecular polarity arises from the unequal distribution of electron density within a molecule. Polar molecules have a dipole moment, a measure of the separation of positive and negative charges. The shape of a molecule plays a crucial role in determining its polarity. Symmetrical molecules, even with polar bonds, can be nonpolar if the bond dipoles cancel each other out.
B. Boiling Point and Melting Point
Intermolecular forces, such as dipole-dipole interactions and hydrogen bonding, influence the boiling and melting points of substances. Molecular shape affects the strength of these intermolecular forces. Molecules with similar molar masses but different shapes can have significantly different boiling and melting points. For example, linear molecules generally have higher boiling points than branched molecules due to stronger intermolecular interactions.
C. Reactivity
Molecular geometry influences a molecule's reactivity by determining the accessibility of its reactive sites. Steric hindrance, the repulsion between atoms or groups of atoms, can affect the rate and selectivity of chemical reactions.
IV. Advanced Examples and Applications
Let's examine some complex examples to reinforce our understanding:
A. SF₆ (Sulfur Hexafluoride)
SF₆ has six electron domains (six bonding pairs), resulting in an octahedral geometry. The molecule is nonpolar due to its symmetrical structure. The sulfur atom undergoes sp³d² hybridization.
B. XeF₄ (Xenon Tetrafluoride)
XeF₄ has six electron domains (four bonding pairs and two lone pairs). The electron domain geometry is octahedral, but the molecular geometry is square planar due to the lone pairs. The xenon atom undergoes sp³d² hybridization.
C. IF₅ (Iodine Pentafluoride)
IF₅ has six electron domains (five bonding pairs and one lone pair). The electron domain geometry is octahedral, and the molecular geometry is square pyramidal due to the lone pair. The iodine atom undergoes sp³d² hybridization.
V. Limitations of VSEPR and Valence Bond Theory
While VSEPR and valence bond theory are powerful tools, they have limitations:
- VSEPR: It's a qualitative model, providing approximate geometries. It doesn't account for subtle deviations in bond angles or the effects of multiple bonding.
- Valence Bond Theory: It simplifies bonding, not fully representing the delocalization of electrons in molecules with multiple bonds.
More advanced theories, like molecular orbital theory, provide a more comprehensive description of bonding and molecular structure.
VI. Conclusion
Understanding the geometrical structure of molecules is crucial for comprehending their properties and reactivity. VSEPR theory offers a straightforward method for predicting molecular shapes, while valence bond theory and hybridization provide a deeper understanding of the underlying bonding mechanisms. By mastering these concepts and applying them to various examples, we gain a robust foundation in chemical bonding and molecular structure, enabling us to predict and interpret the behavior of molecules in diverse chemical systems. The applications of these theories extend far beyond basic chemistry, impacting fields such as materials science, biochemistry, and pharmaceutical research. Further exploration of more advanced theories, like molecular orbital theory, will provide an even more nuanced and comprehensive understanding of the intricate world of molecular structure and bonding.
Latest Posts
Latest Posts
-
Which Of The Following Structures Has The R Configuration
Apr 27, 2025
-
To An Economist Total Costs Include
Apr 27, 2025
-
Steroid Hormone Receptors In Animals Tend To Be
Apr 27, 2025
-
A Product Has Utility If It
Apr 27, 2025
-
A Conglomerate Is A Corporation That
Apr 27, 2025
Related Post
Thank you for visiting our website which covers about Advance Study Assignment The Geometrical Structure Of Molecules . We hope the information provided has been useful to you. Feel free to contact us if you have any questions or need further assistance. See you next time and don't miss to bookmark.