Add Curved Arrows To The Reactants In This Reaction
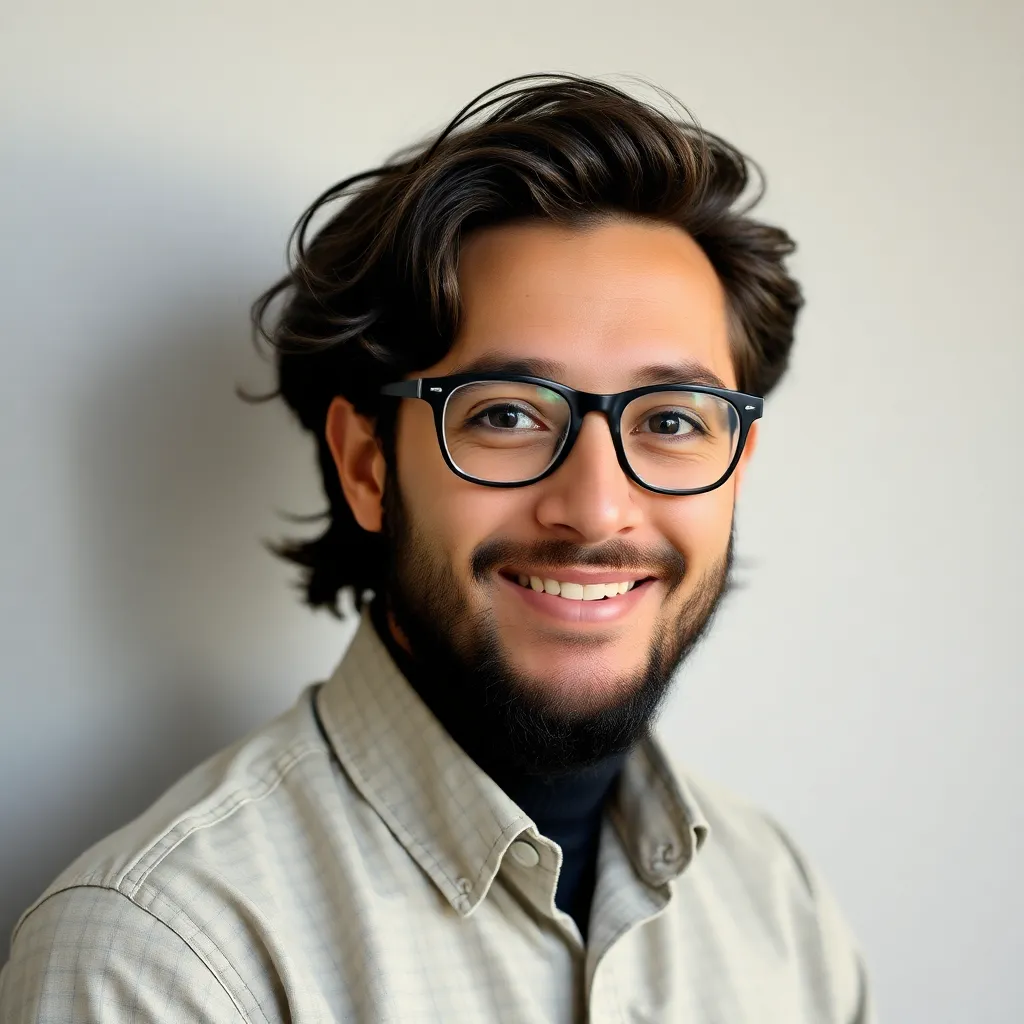
Holbox
May 08, 2025 · 5 min read
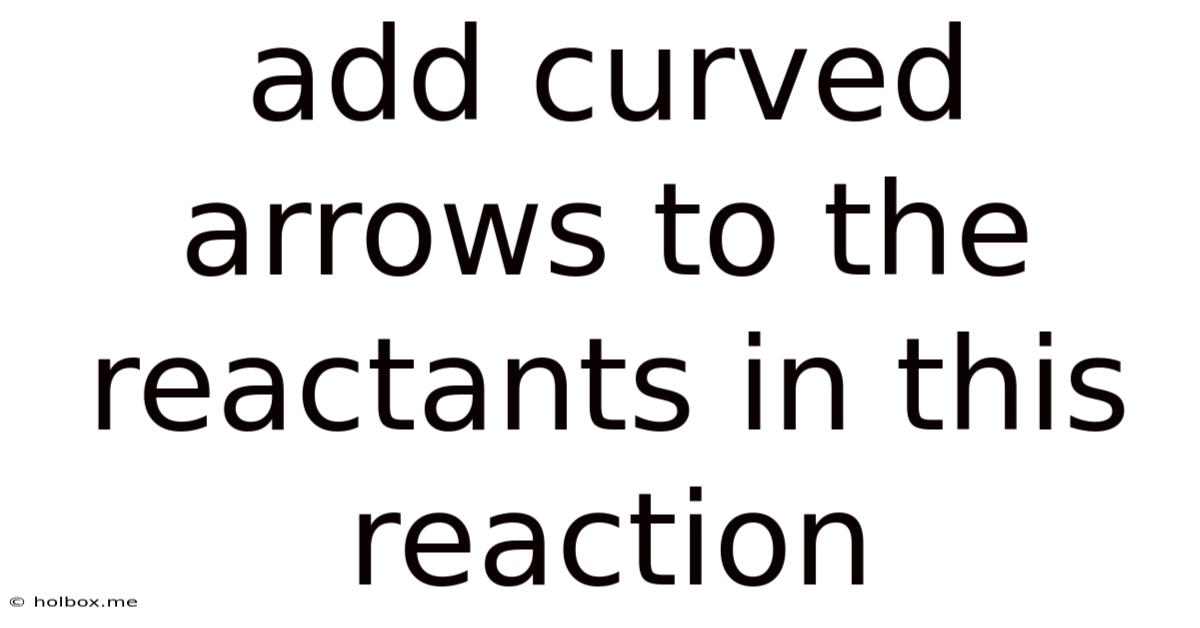
Table of Contents
- Add Curved Arrows To The Reactants In This Reaction
- Table of Contents
- Adding Curved Arrows to Reactants: A Comprehensive Guide to Reaction Mechanisms
- Understanding the Fundamentals of Curved Arrows
- The Arrow's Tale: Electron Movement
- Types of Electron Movement:
- Key Rules for Drawing Curved Arrows:
- Practical Examples: Adding Curved Arrows to Different Reaction Types
- 1. SN1 Reaction (Unimolecular Nucleophilic Substitution):
- 2. SN2 Reaction (Bimolecular Nucleophilic Substitution):
- 3. E1 Reaction (Unimolecular Elimination):
- 4. E2 Reaction (Bimolecular Elimination):
- Advanced Considerations and Practice
- Latest Posts
- Latest Posts
- Related Post
Adding Curved Arrows to Reactants: A Comprehensive Guide to Reaction Mechanisms
Understanding reaction mechanisms is fundamental to organic chemistry. Curved arrows, also known as curly arrows, are the visual language we use to depict the movement of electrons during a reaction. Mastering the art of drawing curved arrows accurately and meaningfully is crucial for predicting reaction products and understanding the underlying processes. This comprehensive guide will delve into the intricacies of adding curved arrows to reactants, covering various reaction types and providing practical examples.
Understanding the Fundamentals of Curved Arrows
Before we dive into specific examples, let's establish the core principles governing the use of curved arrows:
The Arrow's Tale: Electron Movement
Each curved arrow represents the movement of a pair of electrons. The arrow's tail originates from the source of the electrons (often a lone pair, a pi bond, or a sigma bond), while the arrowhead points to where these electrons end up (often forming a new bond, a lone pair, or breaking a bond).
Types of Electron Movement:
-
Nucleophilic Attack: An electron-rich species (nucleophile) donates a pair of electrons to an electron-deficient species (electrophile). The arrow starts from the nucleophile's lone pair or pi bond and points towards the electrophilic atom.
-
Proton Transfer (Acid-Base Reactions): A proton (H⁺) is transferred from an acid to a base. The arrow starts from the lone pair of the base and points towards the proton of the acid. A second arrow shows the breaking of the O-H bond, with the electrons going to the oxygen atom.
-
Leaving Group Departure: A leaving group departs taking a pair of electrons with it. The arrow starts from the bond between the leaving group and the molecule and points toward the leaving group.
-
Radical Reactions: These involve the movement of single electrons, represented by a half-headed arrow (a single-barb arrow). We won't delve deeply into radical reactions here, but understanding this distinction is important for broader chemistry knowledge.
Key Rules for Drawing Curved Arrows:
-
One Arrow per Electron Pair: Each arrow must represent the movement of only one pair of electrons.
-
Conservation of Charge: The overall charge of the reactants must equal the overall charge of the products. The movement of electrons dictates the change in charge.
-
Formal Charge: Always keep track of formal charges on atoms throughout the reaction mechanism. This helps ensure you've accounted for all electrons and are depicting the correct movement.
Practical Examples: Adding Curved Arrows to Different Reaction Types
Let's illustrate the application of curved arrows with diverse examples:
1. SN1 Reaction (Unimolecular Nucleophilic Substitution):
The SN1 reaction involves a two-step mechanism. Let's consider the reaction of tert-butyl bromide with water:
(CH₃)₃CBr + H₂O → (CH₃)₃COH + HBr
Step 1: Carbocation Formation
Here, the C-Br bond breaks heterolytically. The electrons from the bond move to the bromine atom, which departs as a bromide ion (Br⁻). A carbocation intermediate is formed.
(CH₃)₃C—Br ----> (CH₃)₃C⁺ + Br⁻
|
^
|
Curved Arrow showing bond cleavage and electron movement towards Br
Step 2: Nucleophilic Attack
A water molecule, acting as a nucleophile, attacks the carbocation. A lone pair on the oxygen atom of water forms a new bond with the carbocation.
(CH₃)₃C⁺ + H₂O ----> (CH₃)₃C—OH₂⁺
| |
^ ^
| |
Arrow shows electron pair from O attacking the carbocation.
Step 3: Deprotonation
Finally, a base (usually another water molecule) removes a proton from the oxonium ion, forming the alcohol product and regenerating the hydronium ion (H₃O⁺).
(CH₃)₃C—OH₂⁺ + H₂O ----> (CH₃)₃C—OH + H₃O⁺
|
^
Arrow shows proton transfer
2. SN2 Reaction (Bimolecular Nucleophilic Substitution):
SN2 reactions are concerted, meaning bond breaking and bond formation occur simultaneously in a single step. Let's consider the reaction of methyl bromide with hydroxide ion:
CH₃Br + OH⁻ → CH₃OH + Br⁻
In this reaction, the hydroxide ion attacks the methyl bromide from the backside (backside attack). Simultaneously, the C-Br bond breaks, and the electrons move to the bromine atom.
OH⁻ + CH₃—Br ----> CH₃—OH + Br⁻
| | |
^ ^ ^
| | |
Arrow shows nucleophilic attack. Arrow shows bond cleavage.
3. E1 Reaction (Unimolecular Elimination):
E1 reactions also proceed through a carbocation intermediate. Let's examine the dehydration of tert-butyl alcohol:
(CH₃)₃COH → (CH₃)₂C=CH₂ + H₂O
Step 1: Protonation
First, the alcohol is protonated by an acid, making it a better leaving group.
(CH₃)₃C—OH + H⁺ ----> (CH₃)₃C—OH₂⁺
| |
^ ^
Arrow shows proton transfer
Step 2: Carbocation Formation
The protonated alcohol loses a water molecule, forming a carbocation.
(CH₃)₃C—OH₂⁺ ----> (CH₃)₃C⁺ + H₂O
|
^
Arrow shows bond cleavage
Step 3: Deprotonation
Finally, a base removes a proton from one of the methyl groups, forming the alkene.
(CH₃)₃C⁺ ----> (CH₃)₂C=CH₂ + H⁺
| |
^ ^
Arrow shows proton removal, forming double bond.
4. E2 Reaction (Bimolecular Elimination):
E2 reactions are concerted, like SN2 reactions. Let's consider the dehydrohalogenation of ethyl bromide:
CH₃CH₂Br + OH⁻ → CH₂=CH₂ + H₂O + Br⁻
In this reaction, the hydroxide ion acts as a base, abstracting a proton from the beta-carbon. Simultaneously, the C-Br bond breaks, forming the double bond.
OH⁻ + CH₃—CH₂—Br ----> CH₂=CH₂ + H₂O + Br⁻
| | |
^ ^ ^
| | |
Arrow shows proton removal. Arrow shows bond cleavage and double bond formation.
Advanced Considerations and Practice
Mastering curved arrows requires consistent practice. Work through numerous examples in your textbook or online resources. Pay close attention to:
-
Resonance Structures: Curved arrows are crucial for depicting resonance, showing the movement of electrons within a conjugated system.
-
Pericyclic Reactions: Reactions like Diels-Alder and electrocyclic reactions involve concerted cyclic movement of electrons, demanding careful application of curved arrows to illustrate the pericyclic process.
-
Redox Reactions: While less directly related to the movement of electron pairs, curved arrows can also help visualize the transfer of electrons in oxidation-reduction reactions, by depicting the movement of electrons in a redox couple.
By consistently applying these principles and practicing with various reaction types, you'll gain confidence in drawing accurate and meaningful curved arrows, significantly enhancing your understanding of organic reaction mechanisms. Remember, proficiency in this visual language is a cornerstone of mastering organic chemistry.
Latest Posts
Latest Posts
-
How Many Inches In 38 Cm
May 19, 2025
-
What Is 83 Kg In Lbs
May 19, 2025
-
How Much Is 135 Kg In Pounds
May 19, 2025
-
How Many Days Is 127 Hours
May 19, 2025
-
How Many Kilograms Is 66 Pounds
May 19, 2025
Related Post
Thank you for visiting our website which covers about Add Curved Arrows To The Reactants In This Reaction . We hope the information provided has been useful to you. Feel free to contact us if you have any questions or need further assistance. See you next time and don't miss to bookmark.